- Journal List
- GeroScience
- v.42(4); 2020 Aug
- PMC7394991

Perinatal diet influences health and survival in a mouse model of leukemia

Dushani L. Palliyaguru
1Translational Gerontology Branch, National Institute on Aging, NIH, 251 Bayview Blvd, Suite 100, Baltimore, MD 21224 USA
Annamaria L. Rudderow
1Translational Gerontology Branch, National Institute on Aging, NIH, 251 Bayview Blvd, Suite 100, Baltimore, MD 21224 USA
Alex M. Sossong
1Translational Gerontology Branch, National Institute on Aging, NIH, 251 Bayview Blvd, Suite 100, Baltimore, MD 21224 USA
Kaitlyn N. Lewis
1Translational Gerontology Branch, National Institute on Aging, NIH, 251 Bayview Blvd, Suite 100, Baltimore, MD 21224 USA
Caitlin Younts
1Translational Gerontology Branch, National Institute on Aging, NIH, 251 Bayview Blvd, Suite 100, Baltimore, MD 21224 USA
Kevin J. Pearson
2Department of Pharmacology and Nutritional Sciences, University of Kentucky College of Medicine, Lexington, KY USA
Michel Bernier
1Translational Gerontology Branch, National Institute on Aging, NIH, 251 Bayview Blvd, Suite 100, Baltimore, MD 21224 USA
Rafael de Cabo
1Translational Gerontology Branch, National Institute on Aging, NIH, 251 Bayview Blvd, Suite 100, Baltimore, MD 21224 USA

Associated Data
- Data Availability Statement
Not applicable.
Not applicable.
Abstract
The goal of the current study was to determine the role of maternal diet in the perinatal period on the health and survival of the offspring. AKR/J mice, a model described to be susceptible to leukemia development, was used where females were maintained on either standard diet (SD), high sucrose diet, Western diet, or calorie restriction (CR) as they were mated with SD-fed males. Body weights, pregnancy rates, litter size, and litter survival were used as markers of successful pregnancy and pup health. Data indicated that maternal diet had significant effects on litter size, early pup survival, and early pup body weights. As pups matured, the makeup of their respective maternal diet was a predictor of adult metabolic health and survival. Overall, these results suggest that perinatal maternal diet is an important determinant of the health and survival of the offspring and that these effects continue well into adulthood, strongly correlating with lifespan.
Introduction
Diet-induced obesity is one of the most notable public health concerns that leads to increased risk of type 2 diabetes and cardiovascular disease (Must et al. 1999). Poor quality diet is a prominent but modifiable risk factor for obesity and many chronic illnesses (Hall and Guo 2017). Prolonged and repeated consumption of high amounts of refined sugars, salt, and fats often leads to adverse health outcomes (Myles 2014). Specifically, consumption of high levels of carbohydrates, in the form of sucrose or fructose, has detrimental health effects (Kroemer et al. 2018; McDonald 1995). Consumption of a Western diet is often associated with low physical activity (Bortolin et al. 2018), suggesting that the quality and food sources of nutrients may influence a variety of physiological parameters and behaviors. Conversely, caloric restriction (CR) without malnutrition has been shown to improve cardiovascular parameters and body composition in humans (Stekovic et al. 2019), indicating that dietary composition as well as diet quantity plays an important role in outcomes related to human health.
Nutrition at various life stages can have lasting effects on health (Tarry-Adkins and Ozanne 2014). In humans, the nutritional status of mothers significantly contributes to birthweight and is associated with the offspring’s risk for future health complications (Danielewicz et al. 2017). Thus, maternal nutrition has been proposed as an opportunity to prevent type 2 diabetes (Silva-Zolezzi et al. 2017). Conversely, controlling maternal caloric intake may prevent gestational diabetes and excessive weight gain during pregnancy but strict guidelines need to be followed in order to avoid malnutrition, which continues to be a significant global public health issue (Phelps and Metzger 1992). Children born in low-income countries are more likely to not reach their full developmental potential due to maternal and early life nutrient deficiencies resulting from malnutrition (Symington et al. 2018).
Animal models provide valuable tools to study maternal exposures, particularly since studies involving pregnant women pose specific challenges (Dominguez et al. 2012). A 10-week maternal consumption of a Western diet (40% kcal from fat and 43% from carbohydrates, mostly sucrose) during pregnancy increased mortality rate in neonatal mice (Perez and DiPatrizio 2018). Interestingly, exposure to high-fat feeding before and not during pregnancy, significantly reduced fetal and neonatal blood glucose concentrations by enhancing fetal pancreatic β cell development (Qiao et al. 2019). Female offspring of mothers that were fed a high-sucrose diet showed insulin resistance, obesity, and hypertension (Samuelsson et al. 2013). Offspring behavior can also be programmed by maternal high-fat diet feeding during the perinatal period (Sullivan et al. 2014). On the other hand, implementation of early life CR-like strategies including litter size manipulation, crowded litters, and lower maternal milk production has been shown to enhance pup lifespan (Davis et al. 2016). Whether maternal exposure to various diet types during fetal development can reprogram health leading to modulations in survival of the offspring later in life is currently an area that has not been explored in depth.
The AKR/J mouse strain has a relatively short lifespan of about 326 days for males and 276 days for females, and is susceptible to developing leukemia around 6 months of age (Kaptzan et al. 2004). Previous studies have shown that AKR mice are responsive to CR and have lower incidence of leukemia leading to better survival compared to ad-libitum-fed controls (Shields et al. 1991). Although AKR/J mice develop obesity in response to high-fat diet (West et al. 1994), it is unclear whether the manipulation of maternal diets can influence the health and lifespan of their offspring. Mouse maternal diets affect postnatal growth and expression of proteins involved in lifespan regulation (Chen et al. 2009), but reports examining the effect on progeny lifespan itself are limited. Some studies suggest that response to CR is not universal in all mouse strains and the beneficial outcomes are dependent on interactions between degree of CR, diet composition, sex and genetic background, as well as age of onset (Liao et al. 2010; Ingram and de Cabo 2017; Mitchell et al. 2016, 2019). Furthermore, age of onset of CR plays an important role in health and lifespan benefits (Vaughan et al. 2017). Thus, the exact effects of maternal diets, including CR, on the healthspan and lifespan of offspring remain largely unknown. The goal of the present study was to perform a side-by-side comparison of the effect of various types of diet given to female AKR mice to address this gap in knowledge.
Materials and methods
Animals and husbandry
A total of 80 male and 160 female AKR/J inbred strain mice were purchased at 4 weeks of age from The Jackson Laboratory (#000648, Bar Harbor, ME) and housed at the National Institute on Aging animal facilities (Baltimore, MD). All mice were acclimated for at least 1 month to laboratory chow [2018 Teklad Global 18% Protein Rodent Diet, Envigo, (originally Harlan Teklad Global Diets) Madison, WI] upon arrival. They had unlimited access to food and filtered Baltimore city tap water. Cages were changed on a weekly basis, with spot changes as needed. Animal rooms were maintained at 22.2 ± 1 °C and 30–70% humidity on a 12-h day/light cycle, with the lights turned on from 6:00 AM–6:00 PM each day. Animals were inspected twice daily for health issues and veterinary care was provided as needed. Animal protocols were approved by the Animal Care and Use Committee (ACUC) of the National Institute on Aging, National Institutes of Health.
Mating and pregnancy
Based on concerns from the Interventions Testing Program (Nadon et al. 2008), pups from the second pregnancy were used for the experiment to avoid external factors, such as poor maternal care of the first litter. For the initiation of the study and generation of first litter, female mice were housed two per cage and were started on the experimental diets for 1 week before introducing one male mouse per cage for mating. Dams ate the respective diets followed by a period of normal chow during gestation and nursing of first litter. After removal of the first litter, the females were placed on their respective diets for 7–10 days prior to returning to the male (Fig. 1a). After obvious signs of pregnancy, males and females were separated into individual cages. Females remained on the experimental diet throughout the breeding, gestation, and nursing period, until the pups reached 2 weeks of age, at which point the maternal diet was switched to standard mouse chow diet (2018 Teklad Global 18% Protein Rodent diet) and fed ad libitum. After an additional 1-week nursing period, the offspring were weaned into new cages and were fed standard chow for the rest of their lives (Fig. 1a). Food consumption and bodyweight of the pups were measured every week.
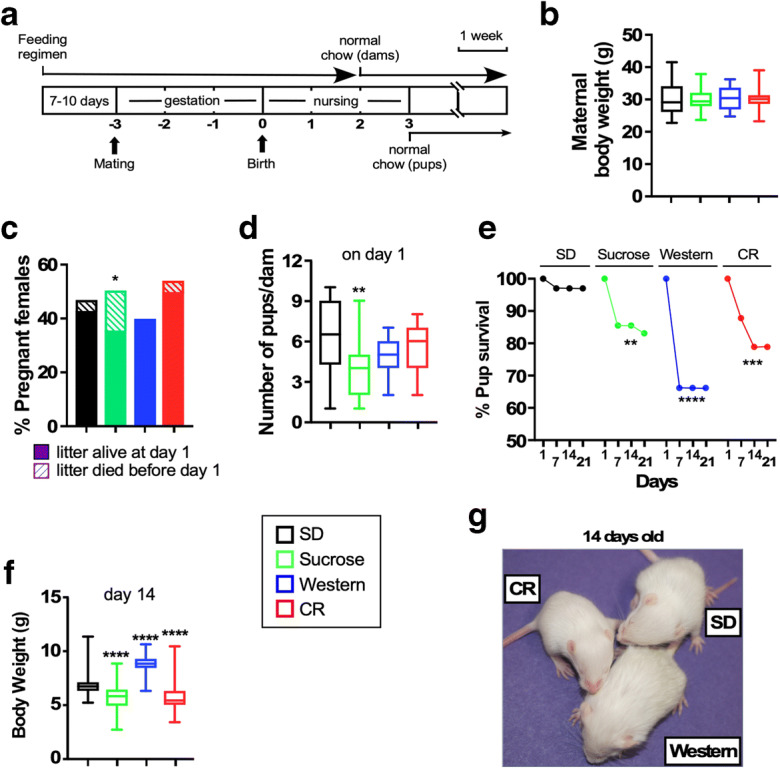
Effects of maternal dietary composition on litter size, early pup survival and bodyweight trajectories. a Schematic of study design. Females were started on the diets 7–10 days prior to mating and were maintained on the diets through mating, gestation, and up until 2 weeks after giving birth. Mothers were switched to a normal chow at this point. After nursing for 3 weeks, pups were weaned to a normal chow for the reminder of their lives. Scale bar, 1 week (b) Maternal body weights at baseline. n = 25–28 mice per dietary group. c Percentage of females that became pregnant. Stacked bars represent the fraction of litters that survived (filled bars) at day 1 and those that did not (hatched bars). Statistical analysis was conducted by Fisher’s exact test. *p < 0.05. d Number of pups per dam at day 1. **, p < 0.01 versus SD group. e Percentage of pups that survived up to 21 days after birth. Number of pups whose dams were on either SD (n = 100), high sucrose diet (83), Western diet (74), or CR (123). **, ***, ****p < 0.01, 0.001, 0.0001 versus pup survival at day 1. f Bodyweights of pups at day 14 post-birth.****p < 0.0001 versus SD. g Representative images of 14-day-old pups. Offspring of dams fed sucrose diet had a body size that was approximately between SD and CR (not shown)
Dietary assignments
For the dietary assignments, females were randomly assigned to four experimental diet groups that consisted of standard laboratory chow (Formulab diet #5008 from Purina; 26.8% protein, 16.7% fat, and 56.5% carbohydrates, 2.6% sucrose), high sucrose diet (#58R1 from Purina [formerly known as KT231, now called 5TUS]; 15% protein, 10.6% fat, and 73.6% carbohydrates, 60% sucrose), Western diet (#5342 from Purina; 15.8% protein, 40% fat, and 44.2% carbohydrates), and a calorie restricted (CR) diet group, which was given 15% less calories than the ad libitum-fed standard chow group.
Survival study
Animals were assessed twice daily for health issues. Death was recorded for each animal. Moribund animals were euthanized, and every animal found dead or euthanized was necropsied. The criteria for euthanasia was based on an independent assessment by a veterinarian according to AAALAC guidelines and only cases where the condition of the animal was considered incompatible with continued survival are represented as deaths in the curves. Histopathological characterization was not conducted during this study so cause of death is unknown.
Body composition
Lean, fat, and fluid mass in live mice were measured by nuclear magnetic resonance (NMR) using the Minispec LF90 (Bruker Optics, Billerica, MA) at the indicated times.
Fasting blood glucose
All mice were fasted for 18 h (from 3:30 PM–9:30 AM) prior to blood collection. Fasting blood glucose was measured in fresh whole blood using a handheld instant glucose meter Bayer Breeze2 (Bayer, Mishawaka, IN).
Rotarod
Motorfunction capacity of mice was tested using the accelerating rotarod test. After a habituation trial at a constant speed of 4 rpm for 1 min before the first trial, mice were tested at the same time every day. On the same day, mice were given three trials on the accelerating rotarod, during which the rotarod accelerated from 4 to 40 rpm over a period of 5 min. Each trial was separated by a 30-min rest period. The latency to fall was recorded and averaged over the three trials. All mice were acclimated for 15 min before testing.
Statistical analysis
No statistical methods were used to predetermine sample size. Investigators were not blinded to allocation during experiments and outcome assessments. Mortality during the survival study was assessed using the log rank test to compare the differences in Kaplan-Meier survival curves between a given dietary group compared to control. Maximal lifespan was defined as the 10th percentile of mice still alive. Differences between control and other dietary groups were tested by either two-tailed Student’s t test using Excel 2019 (Microsoft Corp., Redmond, WA, USA) or one-way ANOVA followed by Dunnett’s multiple comparison using GraphPad Prism v. 6 (GraphPad Software, Inc., La Jolla, CA). D’Agostino and Pearson normality test was performed for all datasets. For data that was not normally distributed, Kruskal-Wallis ANOVA test was used. Litter survival was analyzed by Fisher’s exact test. In all cases, p < 0.05 was considered statistically significant and only data that fit this criterion was depicted using asterisks. Data are expressed as mean ± SEM for the trajectory of food intake, whereas all other data were represented as box plots unless otherwise indicated.
Results and discussion
Maternal body weight measured immediately after giving birth was not significantly impacted by the diet type or dietary regimen (Fig. 1b). Approximately, 40–55% of females became pregnant where sucrose-fed females showed a significantly different number of litters that survived until day 1 compared to SD (Fig. 1c). Given that the viability of litters is an important determinant of early survivability, the litter size per dam in the four maternal diet groups was quantified. On day 1 of giving birth, the number of pups per litter was significantly lower when dams were fed a high sucrose diet and also trended lower in the Western diet and 15% CR groups compared to the SD-fed dams (Fig. 1d). A progressive increase in pup mortality was observed postnatally within the first 21 days when nursed by dams that were fed Western diet > CR > high sucrose > SD. At 21 days, survival was ~ 65% for the Western group, ~ 75% for the CR group, and ~ 80% for the sucrose group, compared to 95% survival for the SD group (Fig. 1e). At 14 days from birth, pups showed significantly different body weights compared to SD where pups of sucrose-fed and CR dams showed lower body weights while pups of Western-fed dams showed higher body weights (Fig. 1f). Figure Figure1g1g depicts representative images of 14-day-old pups nursed by dams fed SD, Western diet, or CR. Offspring of dams fed sucrose diet generally had a body size that was between SD and CR (image not shown). Pups of dams fed a Western diet were larger in body size, had a scruffier coat and weighed more than SD controls, similar to observations made in previous reports (Du et al. 2012) whereas pups from the other groups were more or less comparable to SD.
Given that maternal diets can have lasting effects on lifespan and health of offspring, we examined the impact of maternal diet composition on several health markers. As anticipated, offspring from dams fed Western diet had a trend to gain more body weight regardless of sex, while those from CR-fed dams showed a trend for least body weight gain during the first 6 months of life (Fig. 2a). However, the body weight differences between groups were not statistically significant. The body weight trajectory of pups of dams maintained on high sucrose trended lower than pups nursed by dams that were maintained on SD (Fig. 2a). Maternal diets had minimal effects on the amount of food consumed by the offspring during the first 4 weeks after weaning (Fig. 2b). Fasting blood glucose (FBG) levels were measured in 15–17-week-old offspring mice and found to be comparable between all four experimental groups (Fig. 2c). Lastly, NMR spectroscopy was employed to measure body composition in 14–16 week-old offspring. Sex-specific differences in percent fat mass and associated lean-to-fat ratio were observed, with male offspring of dams maintained on high sucrose diet or CR being significantly leaner than males of SD-fed dams (Fig. 2d, e). No alterations in these morphological measures were noted in female progeny (Figs. 2d, e). By and large, there was little effect of maternal diet on motor coordination in the progeny regardless of sex on the accelerating rotarod. However, female progeny from Western diet-fed mothers showed a significant decrease in performance, which may be related to the trend toward increased body weight in this group (Fig. 2f).
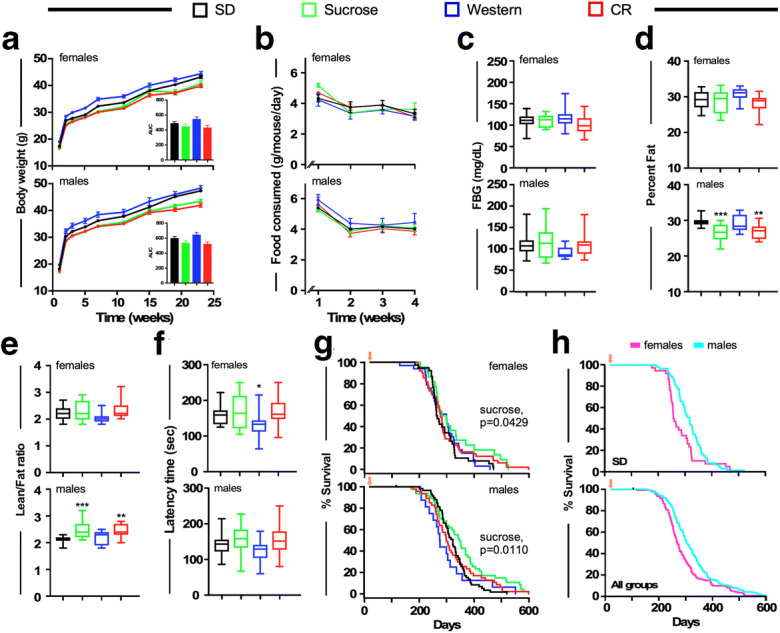
Implications of maternal diet composition on markers of health and survival of the pups. a Body weight trajectories over 25 weeks. Inset, area under the curve (AUC). b Trajectories of food consumption for both female and male pups over a 4-week period. c Fasting blood glucose (FBG) levels in 15–17-weeks-old female (n = 14–32) and male (n = 11–49) pups. d Percent fat in 14–16-weeks-old female (n = 14–15) and male (n = 11–15) pups as assessed by nuclear magnetic resonance spectroscopy (NMR). e Lean-to-fat ratio. f Latency to fall from the accelerating rotarod. Data are represented as whisker plots (c-f) and analyzed using one-way ANOVA with Dunnett’s post-test. *,**, ***p < 0.05, 0.01, and 0.001 vs. SD. g Kaplan-Meier survival curves for female and male offspring from dams that were maintained on either SD [n = 38(F)-59(M)], high sucrose diet [n = 22(F)-47(M)], Western diet [n = 33(F)-16(M)], or 15% CR [n = 49(F)-47(M)]. h Kaplan-Meier survival curves for female and male offspring on SD (upper panel) or after combining all groups (lower panel). F, female; M, male. See Table Table11 for additional information. g, h Orange arrow represents time at weaning (21 days) when mice were enrolled into the survival analysis
Previous studies have shown that almost all ad libitum-fed AKR mice die from luekemia (Shields et al. 1991). When the effect of maternal diet was investigated, we found notable differences in survival among groups of AKR progeny mice (Fig. 2g). Median survival shown in Table Table11 revealed that all 3 maternal diet groups resulted in higher median lifespan in female progeny (284.5, 293, 271 days for sucrose, Western and CR, respectively) compared to SD controls (257.5 days), while in males the sucrose group showed the highest median survival (343 days) followed by SD (317 days), CR (293 days), and Western (268.5 days) groups. Differences were observed in maximum lifespan as well, which is defined as the remaining 10% survival. In females, the sucrose (485 days) and CR (467 days) groups exhibited an extended maximum lifespan compared to SD (326 days) or Western diet (390 days) (Fig. 2g). Similarly, differences in maximum lifespan were observed in male progeny: sucrose (518 days), CR (472 days), SD (383 days), and Western diet (358 days) (Fig. 2g). Out of these, sucrose females and males showed a significantly enhanced lifespans compared to SD (p = 0.0429 and p = 0.0110, respectively). Significant differences were observed in median lifespan between female and male progeny from SD-fed dams (Fig. 2h, upper panel, and Table Table1)1) and from dams maintained on all four dietary regimens (Fig. 2h, lower panel, and Table Table1).1). It is currently unknown if maternal diet elicits directly or indirectly an alteration in the viral transcriptomic machinery that drives lifespan in this model. Interestingly, compared to observations from Sheilds and colleagues where AKR mice showed a maximum lifespan of ~ 52 weeks (Shields et al. 1991) and other reports on AKR lifespan (~ 44 weeks) (Festing and Blackmore 1971), our AKR mice survived much longer. Differences were also noted where male offspring lived longer than females regardless of the maternal diet. Mechanisms underlying these sex differences are poorly understood and warrant further investigation. Collectively, in both female and male offspring, maternal sucrose-rich diet and CR resulted in median and maximum lifespan extension. Altogether, it would appear that the maternal diet, consumed during the pre and perinatal period has a significant effect on metabolic health measures and lifespan of offspring.
Table 1
Median and maximum survival (days) of progeny mice from dams on different maternal diet compositions or CR. Maximum lifespan is defined as the time when the remaining 10% mice are still alive.
n | Median (days) | Maximum (days) | p value | |
---|---|---|---|---|
SD-F | 38 | 257.5 | 326 | – |
Sucrose-F | 22 | 284.5 | 485 | 0.0429* |
Western-F | 33 | 293 | 390 | 0.8895 |
CR-F | 49 | 271 | 467 | 0.3663 |
SD-M | 59 | 317 | 383 | – |
Sucrose-M | 47 | 343 | 518 | 0.0110* |
Western-M | 16 | 268.5 | 358 | 0.5767 |
CR-M | 47 | 293 | 472 | 0.6609 |
SD-F | 38 | 257.5 | 326 | – |
SD-M | 59 | 317 | 383 | 0.0212* |
ALL-F | 142 | 271 | 403 | – |
ALL-M | 169 | 305 | 457 | 0.0025** |
n number of mice. SD standard diet, CR calorie restriction, ALL combination of the four experimental groups, M males, F females. Statistical significance was determined for entire lifespan by Mantel-Cox test compared to SD control or other control counterpart (M or F)
An unexpected finding of the study is that female and male progeny of both sucrose-fed dams and dams on CR showed enhanced lifespan (both median and maximum lifespan, only former statistically significant). Although the effects of CR on lifespan and health biomarkers have been previously reported by us and others (Mitchell et al. 2018), it is unclear how a maternal diet that is high in sucrose can positively influence health-related outcomes and extend longevity. One possibility is that the consistency, taste, and smell of the sucrose diet is unpalatable for mice, causing them to undergo a CR-like effect (de Cabo lab unpublished observation). Previous reports have indicated that fructose is the main driver of the spike in metabolic disorders in humans (Bray et al. 2004). Complementary to these findings, adult female and male mice fed a diet high in fructose/glucose experienced a decline in survival that was more dramatic than mice fed a diet high in sucrose (Ruff et al. 2015), a phenotype likely attributable to differences in glucose uptake/absorption. Diets low in protein and high in carbohydrates lead to longer lifespans in adult ad libitum-fed mice (Le Couteur et al. 2016). Indeed the method of sucrose delivery has been shown to be an important determinant of health outcomes where liquid sucrose rather than solid sucrose resulted in body weight gain in C57BL/6 mice (Togo et al. 2019). Whether a different sugar type, such as fructose, or a different delivery method would result in alternative lifespan and health outcomes is currently not known and must be investigated in future studies.
Fetal malnutrition, resulting from undernourished mothers, has detrimental effects on the offspring and can derail the trajectory of an otherwise potentially healthy fetus. Undernourished mothers expose the fetus to a lack of nutrients, resulting in low birth weight and increased susceptibility to diseases. Moreover, maternal overnutrition exposes offspring to an excess of nutrients, resulting in high birth weight, susceptibility to diseases, and adverse metabolic phenotypes in the offspring (Sun et al. 2012; Martin-Gronert and Ozanne 2006). In the present study, we attempted to represent conditions across this feeding spectrum. The degree and timing of maternal CR plays an important role in health outcomes in pups. Previous studies have shown that maternal 30% CR leads to fetal hyperphagia, hypertension, and hyperinsulinemia (Vickers et al. 2000) whereas maternal 20% CR causes no differences in litter size or body weight in the offspring (Stone et al. 2019). CR has been shown to elicit age-dependent effects on health (Chen et al. 2019) and therefore, the age at which maternal CR was conducted may directly influence pup health as well. However, the effect of various degrees of dietary exposures including CR, on pups from younger or older mothers was not examined in the present study. The exact mechanisms by which maternal nutrition during early pregnancy adversely affects offspring health and development remain poorly understood; however, epigenetic and developmental reprogramming appears to play a crucial role (Dearden and Ozanne 2015) as evidenced by the fact that undernourished children have a distinct methylation signature (Uchiyama et al. 2018). Various maternal factors such as diet, environmental exposure, and stress are likely to impact offspring health (Preston et al. 2018) through a variety of different pathways including stem cell alteration, gut microbiota, and immunomodulation (Friedman 2018). Prior studies have also shown that CR increases the number of primodial follicles and enhances ovarian reserve (Garcia et al. 2019) which can significantly contribute to early life health. Data from our study showed that maternal diet during the gestational and nursing periods had the most profound long-term implications for offspring lifespan, likely through alteration in molecular landscapes related to survival.
There are some limitations in the present study. First, the AKR mouse model used here has the endogenous AKV murine leukemia retrovirus in all tissues that renders them predisposed to lymphatic leukemia at early ages, and it is unknown how this factors into health and survival matrices. While leukemia incidence was not an endpoint that was quantified per se in the present study, previous reports have shown that 60–70% of mice of this strain develop lymphomas (Chrisp et al. 1996). Whether these findings can be extrapolated into other strains of mice that are more representative of mouse models used in biomedical research studies should be a focus of future research. Second, it is unclear how differences in early pup survival could contribute to the overall survival of each experimental group. Because different numbers of mice were dying post-natally prior to day 21 for each group, a survival bias may have been introduced when monitoring the remaining mice, thus affecting lifespan outcomes. Pup survival can dictate litter size and lead to crowded litters and food restriction (Sadagurski et al. 2015). Third, we did not investigate possible diet-mediated alterations in nutrient uptake or absorption, which could have a profound influence on the ability of macronutrients to enter systemic circulation in the dams and impact whole-body metabolic and physiological responses.
Acknowledgements
The authors would like to especially thank the members of the Translational Gerontology Branch and the Comparative Medicine Section of the National Institute on Aging. We are particularly grateful to Dawn Boyer for their excellent animal care during this study.
Authors’ contributions
All experiments were designed by K.J.P. and R.d.C. Lifespan and whole-body experiments were carried out by A.M.S., K.N.L, C.Y., and K.J.P. Data analysis and interpretation was conducted by D.L.P, A.R., M.B., and R.d.C. The manuscript was written by D.L.P., A.R., M.B., and R.d.C.
Funding information
This work was supported by funding from the Intramural Research Program of the National Institute on Aging/NIH.
Compliance with ethical standards
None to declare.
Not applicable.
Not applicable.
Not applicable.
Footnotes
Publisher’s note
Springer Nature remains neutral with regard to jurisdictional claims in published maps and institutional affiliations.
References
- Bortolin RC, Vargas AR, Gasparotto J, Chaves PR, Schnorr CE, Martinello KB, Silveira AK, Rabelo TK, Gelain DP, Moreira JCF. A new animal diet based on human Western diet is a robust diet-induced obesity model: comparison to high-fat and cafeteria diets in term of metabolic and gut microbiota disruption. Int J Obes. 2018;42(3):525–534. [PubMed] [Google Scholar]
- Bray GA, Nielsen SJ, Popkin BM. Consumption of high-fructose corn syrup in beverages may play a role in the epidemic of obesity. Am J Clin Nutr. 2004;79(4):537–543. [PubMed] [Google Scholar]
- Chen JH, Martin-Gronert MS, Tarry-Adkins J, Ozanne SE. Maternal protein restriction affects postnatal growth and the expression of key proteins involved in lifespan regulation in mice. PLoS One. 2009;4(3):e4950. [PMC free article] [PubMed] [Google Scholar]
- Chen CN, Liao YH, Tsai SC, Thompson LDV. Age-dependent effects of caloric restriction on mTOR and ubiquitin-proteasome pathways in skeletal muscles. Geroscience. 2019;41(6):871–880. [PMC free article] [PubMed] [Google Scholar]
- Chrisp CE, Turke P, Luciano A, Swalwell S, Peterson J, Miller RA. Lifespan and lesions in genetically heterogeneous (four-way cross) mice: a new model for aging research. Vet Pathol. 1996;33(6):735–743. [PubMed] [Google Scholar]
- Danielewicz H, Myszczyszyn G, Dębińska A, Myszkal A, Boznański A, Hirnle L. Diet in pregnancy-more than food. Eur J Pediatr. 2017;176(12):1573–1579. [PMC free article] [PubMed] [Google Scholar]
- Davis K, Chamseddine D, Harper JM. Nutritional limitation in early postnatal life and its effect on aging and longevity in rodents. Exp Gerontol. 2016;86:84–89. [PubMed] [Google Scholar]
- Dearden L, Ozanne SE. Early life origins of metabolic disease: developmental programming of hypothalamic pathways controlling energy homeostasis. Front Neuroendocrinol. 2015;39:3–16. [PubMed] [Google Scholar]
- Dominguez V, et al. Clinical trials during pregnancy: what has been done. Eur J Clin Pharmacol. 2012;68(5):455–458. [PubMed] [Google Scholar]
- Du Y, et al. Maternal western diet causes inflammatory milk and TLR2/4-dependent neonatal toxicity. Genes Dev. 2012;26(12):1306–1311. [PMC free article] [PubMed] [Google Scholar]
- Festing MF, Blackmore DK. Life span of specified-pathogen-free (MRC category 4) mice and rats. Lab Anim. 1971;5(2):179–192. [PubMed] [Google Scholar]
- Friedman JE. Developmental programming of obesity and diabetes in mouse, monkey, and man in 2018: where are we headed? Diabetes. 2018;67(11):2137–2151. [PMC free article] [PubMed] [Google Scholar]
- Garcia DN, Saccon TD, Pradiee J, Rincón JAA, Andrade KRS, Rovani MT, Mondadori RG, Cruz LAX, Barros CC, Masternak MM, Bartke A, Mason JB, Schneider A. Effect of caloric restriction and rapamycin on ovarian aging in mice. Geroscience. 2019;41(4):395–408. [PMC free article] [PubMed] [Google Scholar]
- Hall KD, Guo J. Obesity energetics: body weight regulation and the effects of diet composition. Gastroenterology. 2017;152(7):1718–1727. [PMC free article] [PubMed] [Google Scholar]
- Ingram DK, de Cabo R. Calorie restriction in rodents: caveats to consider. Ageing Res Rev. 2017;39:15–28. [PMC free article] [PubMed] [Google Scholar]
- Kaptzan T, Skutelsky E, Itzhaki O, Sinai J, Michowitz M, Yossipov Y, Schiby G, Leibovici J. Age-dependent differences in the efficacy of cancer immunotherapy in C57BL and AKR mouse strains. Exp Gerontol. 2004;39(7):1035–1048. [PubMed] [Google Scholar]
- Kroemer G, López-Otín C, Madeo F, de Cabo R. Carbotoxicity-noxious effects of carbohydrates. Cell. 2018;175(3):605–614. [PMC free article] [PubMed] [Google Scholar]
- Le Couteur DG, et al. The impact of low-protein high-carbohydrate diets on aging and lifespan. Cell Mol Life Sci. 2016;73(6):1237–1252. [PubMed] [Google Scholar]
- Liao CY, Rikke BA, Johnson TE, Diaz V, Nelson JF. Genetic variation in the murine lifespan response to dietary restriction: from life extension to life shortening. Aging Cell. 2010;9(1):92–95. [PMC free article] [PubMed] [Google Scholar]
- Martin-Gronert MS, Ozanne SE. Maternal nutrition during pregnancy and health of the offspring. Biochem Soc Trans. 2006;34(Pt 5):779–782. [PubMed] [Google Scholar]
- McDonald RB. Influence of dietary sucrose on biological aging. Am J Clin Nutr. 1995;62(1 Suppl):284S–292S. [PubMed] [Google Scholar]
- Mitchell SJ, Madrigal-Matute J, Scheibye-Knudsen M, Fang E, Aon M, González-Reyes JA, Cortassa S, Kaushik S, Gonzalez-Freire M, Patel B, Wahl D, Ali A, Calvo-Rubio M, Burón MI, Guiterrez V, Ward TM, Palacios HH, Cai H, Frederick DW, Hine C, Broeskamp F, Habering L, Dawson J, Beasley TM, Wan J, Ikeno Y, Hubbard G, Becker KG, Zhang Y, Bohr VA, Longo DL, Navas P, Ferrucci L, Sinclair DA, Cohen P, Egan JM, Mitchell JR, Baur JA, Allison DB, Anson RM, Villalba JM, Madeo F, Cuervo AM, Pearson KJ, Ingram DK, Bernier M, de Cabo R. Effects of sex, strain, and energy intake on hallmarks of aging in mice. Cell Metab. 2016;23(6):1093–1112. [PMC free article] [PubMed] [Google Scholar]
- Mitchell SJ, et al. Daily fasting improves health and survival in male mice independent of diet composition and calories. Cell Metab. 2018. [PMC free article] [PubMed]
- Mitchell SJ, Bernier M, Mattison JA, Aon MA, Kaiser TA, Anson RM, Ikeno Y, Anderson RM, Ingram DK, de Cabo R. Daily fasting improves health and survival in male mice independent of diet composition and calories. Cell Metab. 2019;29(1):221–228 e3. [PMC free article] [PubMed] [Google Scholar]
- Must A, Spadano J, Coakley EH, Field AE, Colditz G, Dietz WH. The disease burden associated with overweight and obesity. JAMA. 1999;282(16):1523–1529. [PubMed] [Google Scholar]
- Myles IA. Fast food fever: reviewing the impacts of the Western diet on immunity. Nutr J. 2014;13:61. [PMC free article] [PubMed] [Google Scholar]
- Nadon NL, Strong R, Miller RA, Nelson J, Javors M, Sharp ZD, Peralba JM, Harrison DE. Design of aging intervention studies: the NIA interventions testing program. Age (Dordr) 2008;30(4):187–199. [PMC free article] [PubMed] [Google Scholar]
- Perez PA, DiPatrizio NV. Impact of maternal western diet-induced obesity on offspring mortality and peripheral endocannabinoid system in mice. PLoS One. 2018;13(10):e0205021. [PMC free article] [PubMed] [Google Scholar]
- Phelps RL, Metzger BE. Caloric restriction in gestational diabetes mellitus: when and how much? J Am Coll Nutr. 1992;11(3):259–262. [PubMed] [Google Scholar]
- Preston JD, Reynolds LJ, Pearson KJ. Developmental origins of health span and life span: a mini-review. Gerontology. 2018;64(3):237–245. [PMC free article] [PubMed] [Google Scholar]
- Qiao L, Wattez JS, Lim L, Rozance PJ, Hay WW Jr, Shao J. Prolonged Prepregnant maternal high-fat feeding reduces fetal and neonatal blood glucose concentrations by enhancing fetal beta-cell development in C57BL/6 mice. Diabetes. 2019:db181308. [PMC free article] [PubMed]
- Ruff JS, Hugentobler SA, Suchy AK, Sosa MM, Tanner RE, Hite ME, Morrison LC, Gieng SH, Shigenaga MK, Potts WK. Compared to sucrose, previous consumption of fructose and glucose monosaccharides reduces survival and fitness of female mice. J Nutr. 2015;145(3):434–441. [PMC free article] [PubMed] [Google Scholar]
- Sadagurski, M., et al., Transient early food restriction leads to hypothalamic changes in the long-lived crowded litter female mice. Phys Rep, 2015. 3(4). [PMC free article] [PubMed]
- Samuelsson AM, et al. Sucrose feeding in mouse pregnancy leads to hypertension, and sex-linked obesity and insulin resistance in female offspring. Front Physiol. 2013;4:14. [PMC free article] [PubMed] [Google Scholar]
- Shields BA, Engelman RW, Fukaura Y, Good RA, Day NK. Calorie restriction suppresses subgenomic mink cytopathic focus-forming murine leukemia virus transcription and frequency of genomic expression while impairing lymphoma formation. Proc Natl Acad Sci U S A. 1991;88(24):11138–11142. [PMC free article] [PubMed] [Google Scholar]
- Silva-Zolezzi I, Samuel TM, Spieldenner J. Maternal nutrition: opportunities in the prevention of gestational diabetes. Nutr Rev. 2017;75(suppl 1):32–50. [PMC free article] [PubMed] [Google Scholar]
- Stekovic S, Hofer SJ, Tripolt N, Aon MA, Royer P, Pein L, Stadler JT, Pendl T, Prietl B, Url J, Schroeder S, Tadic J, Eisenberg T, Magnes C, Stumpe M, Zuegner E, Bordag N, Riedl R, Schmidt A, Kolesnik E, Verheyen N, Springer A, Madl T, Sinner F, de Cabo R, Kroemer G, Obermayer-Pietsch B, Dengjel J, Sourij H, Pieber TR, Madeo F. Alternate day fasting improves physiological and molecular markers of aging in healthy, non-obese humans. Cell Metab. 2019;30(3):462–476. [PubMed] [Google Scholar]
- Stone V, Maurmann RM, dal Magro BM, Crestani MS, Hozer RM, Klein CP, et al. Gestational caloric restriction with micronutrients supplementation does not delay development and promotes feeding behavior benefits. Nutr Neurosci. 2019:1–11. [PubMed]
- Sullivan EL, Nousen EK, Chamlou KA. Maternal high fat diet consumption during the perinatal period programs offspring behavior. Physiol Behav. 2014;123:236–242. [PMC free article] [PubMed] [Google Scholar]
- Sun B, Purcell RH, Terrillion CE, Yan J, Moran TH, Tamashiro KLK. Maternal high-fat diet during gestation or suckling differentially affects offspring leptin sensitivity and obesity. Diabetes. 2012;61(11):2833–2841. [PMC free article] [PubMed] [Google Scholar]
- Symington EA, Baumgartner J, Malan L, Zandberg L, Ricci C, Smuts CM. Nutrition during pregnancy and early development (NuPED) in urban South Africa: a study protocol for a prospective cohort. BMC Pregnancy Childbirth. 2018;18(1):308. [PMC free article] [PubMed] [Google Scholar]
- Tarry-Adkins JL, Ozanne SE. The impact of early nutrition on the ageing trajectory. Proc Nutr Soc. 2014;73(2):289–301. [PubMed] [Google Scholar]
- Togo J, Hu S, Li M, Niu C, Speakman JR. Impact of dietary sucrose on adiposity and glucose homeostasis in C57BL/6J mice depends on mode of ingestion: liquid or solid. Mol Metab. 2019;27:22–32. [PMC free article] [PubMed] [Google Scholar]
- Uchiyama R, Kupkova K, Shetty SJ, Linford AS, Pray-Grant MG, Wagar LE, Davis MM, Haque R, Gaultier A, Mayo MW, Grant PA, Petri WA, Jr, Bekiranov S, Auble DT. Histone H3 lysine 4 methylation signature associated with human undernutrition. Proc Natl Acad Sci U S A. 2018;115(48):E11264–E11273. [PMC free article] [PubMed] [Google Scholar]
- Vaughan KL, et al. Caloric restriction study design limitations in rodent and nonhuman primate studies. J Gerontol A Biol Sci Med Sci. 2017;73(1):48–53. [PMC free article] [PubMed] [Google Scholar]
- Vickers MH, Breier BH, Cutfield WS, Hofman PL, Gluckman PD. Fetal origins of hyperphagia, obesity, and hypertension and postnatal amplification by hypercaloric nutrition. Am J Physiol Endocrinol Metab. 2000;279(1):E83–E87. [PubMed] [Google Scholar]
- West DB, Goudey-Lefevre J, York B, Truett GE. Dietary obesity linked to genetic loci on chromosomes 9 and 15 in a polygenic mouse model. J Clin Invest. 1994;94(4):1410–1416. [PMC free article] [PubMed] [Google Scholar]