Highlights
- •An IHF extends lifespan in male rats and flies
- •The IHF decreases the profiles of FFAs in serum and multiple tissues in rats and flies
- •The IHF downregulates anabolism of FFAs and upregulates catabolism of FFAs
- •Decreased FFAs upregulate PPRC1, mediating the effect of IHF on lifespan via PPARG
Summary
Graphical abstract
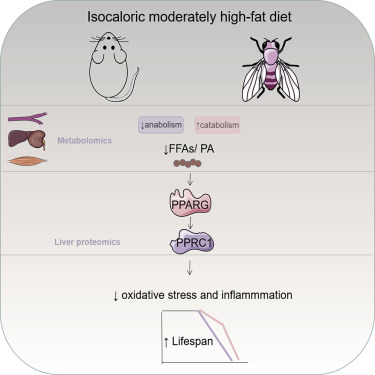
Keywords
Introduction
- Dehghan M.
- Mente A.
- Zhang X.
- Swaminathan S.
- Li W.
- Mohan V.
- Iqbal R.
- Kumar R.
- Wentzel-Viljoen E.
- Rosengren A.
- et al.
Results
IHF prolongs the lifespan and healthspan of rats

Trajectories of biomarkers of aging during the experiment

Profiles of fatty acids in serum, liver, kidney, and muscle
- Inoguchi T.
- Li P.
- Umeda F.
- Yu H.Y.
- Kakimoto M.
- Imamura M.
- Aoki T.
- Etoh T.
- Hashimoto T.
- Naruse M.
- et al.

Liver proteomic changes further reflected the above observations and identified PPRC1 as a candidate target

- Sheedy F.J.
- Grebe A.
- Rayner K.J.
- Kalantari P.
- Ramkhelawon B.
- Carpenter S.B.
- Becker C.E.
- Ediriweera H.N.
- Mullick A.E.
- Golenbock D.T.
- et al.

IHF also prolonged the lifespan in flies

Transgenic RNAi and overexpression flies
Decreased PA upregulates PPRC1, contributing to IHF-mediated changes in lifespan, oxidative stress, and inflammation through PPARG

PPARG/PPRC1 inversely correlates with age detected by human liver
Beta-hydroxybutyrate is a secondary contributor to the IHF-mediated reduction in oxidative stress and inflammation in rats
Discussion
- Dehghan M.
- Mente A.
- Zhang X.
- Swaminathan S.
- Li W.
- Mohan V.
- Iqbal R.
- Kumar R.
- Wentzel-Viljoen E.
- Rosengren A.
- et al.
Conclusions
Limitations of study
STAR★methods
Key resources table
REAGENT or RESOURCE | SOURCE | IDENTIFIER |
---|---|---|
Antibodies | ||
Rabbit monoclonal Anti-Flotillin-1 | Cell Signaling Technology | Cat# 18634; RRID: AB_277304081 |
Rabbit Anti-Fatty Acid Synthase | Cell Signaling Technology | Cat# 3180; RRID: AB_2100796 |
Rabbit monoclonal Anti-Catalase | Cell Signaling Technology | Cat# 14097; RRID: AB_2798391 |
Rabbit monoclonal Anti-GSK-3β | Cell Signaling Technology | Cat# 12456; RRID: AB_26369787 |
Rabbit polyclonal Anti-Acetyl-CoA Carboxylase | Cell Signaling Technology | Cat# 3676; RRID: AB_2219397 |
Mouse monoclonal Anti-β-Actin | Cell Signaling Technology | Cat# 58169; RRID: AB_2750839 |
Rabbit polyclonal Anti-CD36 | Abcam | Cat# ab80978; RRID: AB_1640333 |
Rabbit polyclonal Anti-NCALD | Absin Bioscience | Abs139207 |
Mouse polyclonal Anti-CPTII | Santa Cruz Biotechnology | Sc-377294 |
Rabbit polyclonal Anti-NFκB p65 | WanLeiBio | WL 01273b |
Anti-IL6 Rabbit pAb | WanLeiBio | WL02841 |
Mouse monoclonal Anti-CPT1A | Abcam | Cat# ab128568; RRID: AB_11141632 |
Rabbit monoclonal Anti-PPARγ | Cell Signaling Technology | Cat# 2443; RRID: AB_823598 |
Rabbit monoclonal Anti-PPARγ | WanLeiBio | WL01800 |
Mouse monoclonal Anti-PPRC1 | Santa Cruz Biotechnology | Cat# sc-376431; RRID: AB_11149017 |
Rabbit polyclonal Anti-PPRC1 | OriGene Technologies | Cat# AP53426PU-N; RRID: AB_11145429 |
Anti-Rabbit IgG (Fc), AP Conjugate | Promega | Cat# S3731; RRID: AB_430872 |
Anti-Mouse IgG (H+L), AP Conjugate | Promega | Cat# S3721; RRID: AB_430871 |
Chemicals, peptides, and recombinant proteins | ||
Control diet (custom) | N/A | See Table S1 |
IHF diet (custom) | N/A | See Table S1 |
FHF diet (custom) | N/A | See Table S1 |
Palmitic acid | Sigma-Aldrich | P5585 |
Lipofectamine 2000 Reagent | Invitrogen | Cat#11668027 |
pYr-CMV-Kan2-PPRC1 | Yingrun Biotechnology | HO015062 |
pYr-CMV-Kan2-control | Yingrun Biotechnology | N/A |
PPRC1 siRNA | Santa Cruz Biotechnology | Sc-90572 |
Control siRNA-A | Santa Cruz Biotechnology | Sc-37007 |
Rosiglitazone | MedChemExpress LLC | CAS#122320-73-4 |
Pioglitazone | MedChemExpress LLC | CAS#111025-46-8 |
TRIzol reagent | Invitrogen | Cat#15596026 |
RIPA lysis buffer | Beyotime | P0013C |
Phenylmethanesulfonyl fluoride (PMSF) | Beyotime | ST506 |
Trypsin Gold, Mass Spectrometry Grade | Promega | V5280 |
Rat ACTB Endogenous Reference Genes Primers | Sangon Biotech | B661202-0001 |
Human ACTB Endogenous Reference Genes Primers | Sangon Biotech | B661102-0001 |
Critical commercial assays | ||
Human IL-6 ELISA | Lengton Bioscience | BPE10140 |
Human ROS ELISA | Lengton Bioscience | BPE11725 |
High-Capacity cDNA Reverse Transcription Kit | Applied Biosystems | 4374966 |
Total Nitric Oxide Assay Kit | Beyotime | S0024 |
GSH and GSSG Assay Kit | Beyotime | S0053 |
Lipid Peroxidation MDA Assay Kit | Beyotime | S0131 |
Total Superoxide Dismutase Assay | Beyotime | S0101 |
Mouse/Rat Insulin ELISA Kit | Westang | N/A |
Mouse/Rat IL-6 ELISA Kit | Biotopped | TOPEL02879 |
Mouse/Rat TNF-α ELISA Kit | Biotopped | TOPEL02868 |
Mouse/Rat ROS ELISA Kit | Biocompare | Abx156053 |
Beta-hydroxybutyrate Assay Kit | Sigma-Aldrich | MAK041 |
Deposited data | ||
The proteomics data | This paper | Identifier: PXD022585 |
Experimental models: cell lines | ||
Human hepatocytes (HepG2) cells | Chinese Academy of Science | N/A |
Experimental models: organisms/strains | ||
Wistar rat | Vital River Laboratory Animal Technology Company LTD | N/A |
W1118 | Tsinghua Drosophila Stock Center | THJ0265 |
Dh-PPRC1 RNAi-1 | Tsinghua Drosophila Stock Center | HMS00857 |
Dh-PPRC1 RNAi-2 | Tsinghua Drosophila Stock Center | HMS00858 |
Dh-PPRC1 overexpressions using flySAM Transgenic CRISPRa | Tsinghua Drosophila Stock Center | TH14666S |
UAS-Eip75B overexpressions (genotype: w∗; p {UAS-Eip75B} attP2/ TM6B) | Fungene Biotechnology | N/A |
y[1] w[∗]; P{w[+mC]=Act5C-GAL4} 25FO1/CyO,y[+] | Fungene Biotechnology | N/A |
Ubi-Gal4 | Tsinghua Drosophila Stock Center | TB00152 |
Daughterless Gal4 | Tsinghua Drosophila Stock Center | TB00153 |
Tub Gal4/TM6b, Tb | Tsinghua Drosophila Stock Center | TB00129 |
y v; attP2, y+ | Tsinghua Drosophila Stock Center | TB00072 |
Software and algorithms | ||
Prism 7.0 | GraphPad | http://www.graphpad.com/scientific-software/prism; RRID: SCR_015807 |
SPSS 17.0 | SPSS | 17.0 |
R (version 3.5.1) | R | https://www.r-project.org/ |
DAVID tool | DAVID | http://david.ncifcrf.gov/ |
STRING | STRING | https://string-db.org/ |
Cytoscape (version 3.6.1) | Cytoscape Consortium | https://cytoscape.org/ |
Thermo Scientific Xcalibur 3.1 | Xcalibur | 3.1 |
Other | ||
Shuttle box tests | Taimeng | N/A |
Morris water maze | Taimeng | N/A |
Treadmill | Taimeng | N/A |
ROCHE Modular P800 Biochemical Analyzer | Roche Diagnostics | N/A |
The indirect calorimetry | TSE | N/A |
Calibration capillary | VWR, West Chester, PA | #53432-706 |
GC/MS-MS | Thermo Finnigan | N/A |
Resource availability
Lead contact
Materials availability
Data and code availability
Experimental model and subject details
Animals and diets
Component (g) | Control | IHF | FHF |
---|---|---|---|
Casein | 200.0 | 220.4 | 200.0 |
L-cystine | 3.0 | 3.5 | 3.0 |
Cornstarch | 397.5 | 304.5 | 325.5 |
Dextrinized cornstarch | 132.0 | 114.4 | 114.0 |
Sucrose | 100.0 | 80.0 | 88.0 |
Soybean oil | 70.0 | 70.0 | 70.0 |
Lard | 0.0 | 100.0 | 102.0 |
Mixed minerals | 35.0 | 38.6 | 35.0 |
Mixed vitamins | 10.0 | 11.0 | 10.0 |
Cellulose | 50.0 | 55.1 | 50.0 |
Choline | 2.5 | 2.5 | 2.5 |
1000.0 | 1000.0 | 1000.0 | |
Energy from macronutrients (%) | |||
Carbohydrate | 63.6 | 45.1 | 47.2 |
Protein | 20.5 | 20.3 | 18.2 |
Fat | 15.9 | 34.6 | 34.6 |
Drosophila stocks
Component (g) | Larval media | Control (0%) | IHF (3%) | IHF (7%) | IHF (10%) |
---|---|---|---|---|---|
Agar | 10 | 11 | 11 | 11 | 11 |
Dextrose | 55 | 145 | 103.53 | 48.29 | 1.52 |
Corn meal | 60 | 50 | 50 | 50 | 50 |
Sucrose | 30 | 91 | 64.97 | 30.3 | 9.56 |
Yeast | 25 | 18 | 18 | 18 | 18 |
20% Tegosept | 15 | 15 | 15 | 15 | 15 |
Propionic acid | 3 | 3 | 3 | 3 | 3 |
Lard | 0 | 0 | 30 | 70 | 100 |
Water | 1000ml | 1000ml | 1000ml | 1000ml | 1000ml |
Calorie (cal) | |||||
Carbohydrate | — | 1087.45 | 817.45 | 457.81 | 187.77 |
Protein | — | 75.46 | 75.46 | 75.46 | 75.46 |
Fat | — | 15.50 | 285.50 | 645.50 | 915.50 |
Total calorie | — | 1178.402 | 1178.402 | 1178.762 | 1178.722 |
Cell culture and treatment
Liver sample collection in humans
Method details
Physical performance tests
Collection of blood and tissue
Indirect calorimetry
Proteomics analysis
Protein extraction
- (1)Mix 0.5 ml of lysis buffer 3 (8 M Urea, 40 mM Tris-HCl or TEAB with 1mM PMSF, 2mM EDTA and 10mM DTT, pH 8.5) with 100 mg of liver sample, and place in a 1.5 ml centrifuge tube.
- (2)The mixtures were placed into a TissueLyser with two magnetic beads (diameter 5mm) for 2min at 50Hz to release proteins.
- (3)Centrifugation with 25,000g at 4°C for 20min, and the supernatant was transferred into a new tube.
- (4)Add 10 mM dithiothreitol (DTT) to the supernatant, and 56°C water bath for 1 hour.
- (5)Return to room temperature and then alkylate by 55 mM iodoacetamide (IAM) in the dark at room temperature for 45min.
- (6)Following centrifugation (25,000 g, 4°C, 20 min), the supernatant containing proteins was quantified by Bradford
Quality control of protein extraction
- (1)Protein quantitation with bradford assay. Protein was quantitated according to the manufacturers' protocol.
- (2)SDS-PAGE. After Mixing 30μg proteins with loading buffer in centrifuge tube, heat them at 95°C for 5 minutes to make protein sample. load the protein sample to holes in 12% polyacrylamide gel and then run SDS-PAGE at 120V constant voltage for 120 minutes. After electrophoresis, stain gel with Coomassie Blue for 2 hours, then add destaining solution (40% ethanol and 10% acetic acid) and shake it for 3-5 times, each time for 30 minutes.
Protein digestion
Peptide labeling
Peptide fractionation
HPLC
Mass spectrometer detection
Bioinformatics pipeline
MS/MS raw data
MS/MS ion search and canonical proteomics database
Item | Value |
---|---|
Type of search | MS/MS ion search |
Enzyme | Trypsin |
Fragment mass tolerance | 0.05Da |
Mass values | Monoisotopic |
Variable modifications | Oxidation (M), iTRAQ8plex (Y) |
Peptide mass Tolerance | 20ppm |
Fixed modifications | Carbamidomethyl (C), iTRAQ8plex (N-term), iTRAQ8plex (K) |
Database | I-cxPBT002 (35814 sequences) |
Database_info | Rtus_norvegicus(uniprot,20171117) |
Protein quantification
Item | Value |
---|---|
Quant_peptide | Use All Unique peptide |
Quant_number | At least one unique spetra |
Normalization | VSN |
Protein_Ratio | Weighted average |
Statistical Analysis | Permutation Tests |
GO enrichment and pathway enrichment
Western blot analysis
Quantitative real-time PCR
Primers | Sequences |
---|---|
fruit fly-rpl32-FORWARD | GCACTTCATCCGCCACCAGTC |
fruit fly-rpl32-REVERSE | TGCGCTTGTTCGATCCGTAACC |
fruit fly-dh-PPRC1(spargel)-FORWARD | AAGGAAGCACCAGCACCGAATG |
fruit fly-dh-PPRC1(spargel)-REVERSE | GTGCCTCCAGCGTAGATGAACC |
fruit fly-dh-PPARG(Eip75B)-FORWARD | CTCCACCATCAGCATCAGCATCAG |
fruit fly-dh-PPARG(Eip75B)-REVERSE | CGCCGACTCCAGCAACTTGAC |
fruit fly-whd-FORWARD | TGTTCGACCGCTGCTTGATGATG |
fruit fly-whd-REVERSE | ACTCCTCCCACCAATCCGACAC |
fruit fly-crq-FORWARD | TGCTGAACCATGAAGGCGGAAAG |
fruit fly-crq-REVERSE | GCCACCCGAAGCGTTTGTAGG |
fruit fly-cpt2-FORWARD | GCCGATGGGAAGGTGTTCATAGAC |
fruit fly-cpt2-REVERSE | GCTACTGCTGCTCTGCTGGATG |
fruit fly-acc-FORWARD | AGCAGGCAGGTCAGGTGTGG |
fruit fly-acc-REVERSE | CAATCAGCGGCAACTCCTCTCG |
fruit fly-dorsal-FORWARD | AGCAGCAGCAGCAGCAACAG |
fruit fly-dorsal-REVERSE | TGCCGCCGAGAATTTACTTTCCC |
fruit fly-cat-FORWARD | GAACGGCTATGGCTCGCACAC |
fruit fly-cat-REVERSE | CCAACTGATCGGCGGTCTTCAC |
human-actb- FORWARD | CCTGGCACCCAGCACAAT |
human-actb- REVERSE | GGGCCGGACTCGTCATAC |
human-pparg-FORWARD | AGATCATTTACACAATGCTGGC |
human-pparg-REVERSE | TAAAGTCACCAAAAGGCTTTCG |
human-pprc1-FORWARD | AACTACGGCTTCGTCACTTATC |
human-pprc1-REVERSE | AGATCAGAATAGCTCCTCTTGC |
rat-actb-FORWARD | TGTCACCAACTGGGACGATA |
rat-actb- REVERSE | GGGGTGTTGAAGGTCTCAAA |
rat-ncald-FORWARD | GCCAGATGGACACCAACAGAGATG |
rat-ncald-REVERSE | GCAGGAGACGCACAATGGATGG |
rat-flot1-FORWARD | TGGACATGCTGCTGGAGAAACTG |
rat-flot1-REVERSE | CATGGTTCCGCTTCCGCTAGAC |
rat-gsk3b-FORWARD | CAATCGCACTGTGTAGCCGTCTC |
rat-gsk3b-REVERSE | GGTGTGTCTCGCCCATTTGGTAG |
rat-pprc1-FORWARD | GCTGATGATCTGACACTGCCTGAG |
rat-pprc1-REVERSE | GAATCTGCCGCACGACCACTG |
rat-scot-FORWARD | GGGGTGTGCCTGCTACTTTTCC |
rat-scot-REVERSE | ACACAACCCGAAACCACCAACC |
Food intake measurement in Drosophila
Quantification and statistical analysis
Survival assessment
Pathology examinations and quantification
Blood chemistry measurement
Free fatty acids measurement
Blood beta-hydroxybutyrate levels
Statistical analysis
Acknowledgments
Author contributions
Declaration of interests
Supplemental information
- Document S1. Figures S1–S7 and Tables S1–S4
- Data S1. Original datasets of the results in this manuscript
(A) Pathological records of rats related to Figures 1 and S1.
(B) Survival data of rats related to Figure 1F.
(C) Energy metabolism of rats related to Figures 1H−1O.
(D) Differential protein expressions with regard to oxidative stress and inflammation in the IHF-control/FHF pairwise comparisons related to Figures 4B and 4C
(E) protein-protein interaction differential protein expressions with regard to oxidative stress and inflammation in the IHF-control pairwise comparisons related to Figures 4D and 4F Differential protein expressions with regard to nutrient-sensing and fatty acids metabolism in the IHF-control pairwise comparisons related to Figures S3 and S4.
(G) All proteins in the IHF-control pairwise comparisons related to Figures S3–S5.
(H) All proteins in the FHF-control pairwise comparisons related to Figures S3–S5.
(I) protein-protein interaction for all differential proteins related to Figure S3E.
- Data S2. Proteins and pathways regulated by IHF or FHF versus control, related to Figures 4B, 4C, S3A, and S3B
- Data S3. Statistical analyses of survival curves for flies, related to Figures 6, 7, and S5
References
- CD36 actions in the heart: lipids, calcium, inflammation, repair and more?.Biochim. Biophys. Acta. 2016; 1861: 1442-1449
- Prolonging healthy aging: longevity vitamins and proteins.Proc. Natl. Acad. Sci. USA. 2018; 115: 10836-10844
- PPARgamma2 is a key driver of longevity in the mouse.PLoS Genet. 2009; 5: e1000752
- High-fat diet enhances stemness and tumorigenicity of intestinal progenitors.Nature. 2016; 531: 53-58
- Free fatty acid and glucose metabolism in human aging: evidence for operation of the Randle cycle.Am. J. Physiol. 1994; 266: E501-E509
- The toxic influence of paraquat on hippocampus of mice: involvement of oxidative stress.Neurotoxicology. 2010; 31: 310-316
- Functional aspects of meningeal lymphatics in ageing and Alzheimer's disease.Nature. 2018; 560: 185-191
- Fat, calories, and cancer.Cancer Res. 2016; 76: 509-510
- Associations of fats and carbohydrate intake with cardiovascular disease and mortality in 18 countries from five continents (PURE): a prospective cohort study.Lancet. 2017; 390: 2050-2062
- The ProteomeXchange Consortium in 2020: enabling 'big data' approaches in proteomics.Nucleic Acids Res. 2020; 48: D1145-D1152
- The capillary feeder assay measures food intake in Drosophila melanogaster.J. Vis. Exp. 2017; 121: 55024
- The functions of PPARs in aging and longevity.PPAR Res. 2007; 2007: 39654
- Reprogramming of energy metabolism as a driver of aging.Oncotarget. 2016; 7: 15410-15420
- Promoting health and longevity through diet: from model organisms to humans.Cell. 2015; 161: 106-118
- Extending healthy life span--from yeast to humans.Science. 2010; 328: 321-326
- Saturated fatty acid metabolism is key link between cell division, cancer, and senescence in cellular and whole organism aging.Age (Dordr.). 2010; 32: 231-237
- Fatty acid desaturation links germ cell loss to longevity through NHR-80/HNF4 in C. elegans.PLoS Biol. 2011; 9: e1000599
- Regulatory T cells expressing PPAR-gamma control inflammation in obesity.Cell Metab. 2012; 16: 4-6
- Mono-unsaturated fatty acids link H3K4me3 modifiers to C. elegans lifespan.Nature. 2017; 544: 185-190
- Time-restricted feeding without reducing caloric intake prevents metabolic diseases in mice fed a high-fat diet.Cell Metab. 2012; 15: 848-860
- Reduced expression of MYC increases longevity and enhances healthspan.Cell. 2015; 160: 477-488
- Vitamin D deficiency: a worldwide problem with health consequences.Am. J. Clin. Nutr. 2008; 87: 1080S-1086S
- High glucose level and free fatty acid stimulate reactive oxygen species production through protein kinase C--dependent activation of NAD(P)H oxidase in cultured vascular cells.Diabetes. 2000; 49: 1939-1945
- The effects of ovariectomy and lifelong high-fat diet consumption on body weight, appetite, and lifespan in female rats.Horm. Behav. 2018; 97: 25-30
- The role of lipid metabolism in aging, lifespan regulation, and age-related disease.Aging Cell. 2019; 18: e13048
- Myristoylation of Src kinase mediates Src-induced and high-fat diet-accelerated prostate tumor progression in mice.J. Biol. Chem. 2017; 292: 18422-18433
- Effects of high-fat diet on incidence of spontaneous tumors in Wistar rats.Nutr. Cancer. 1993; 19: 99-110
- High-fat diet fuels prostate cancer progression by rewiring the metabolome and amplifying the MYC program.Nat. Commun. 2019; 10: 4358
- SREBP and MDT-15 protect C. elegans from glucose-induced accelerated aging by preventing accumulation of saturated fat.Genes Dev. 2015; 29: 2490-2503
- The effect of caffeine and albuterol on body composition and metabolic rate.Obesity (Silver Spring). 2015; 23: 1830-1835
- Targeted metabolomics analysis reveals the association between maternal folic acid supplementation and fatty acids and amino acids profiles in rat pups.J. Chromatogr. B. 2018; 1090: 101-109
- Interventions to slow aging in humans: are we ready?.Aging Cell. 2015; 14: 497-510
- The hallmarks of aging.Cell. 2013; 153: 1194-1217
- Mechanisms preserving insulin action during high dietary fat intake.Cell Metab. 2019; 29: 50-63.e54
- Vitamin D supplements and prevention of cancer and cardiovascular disease.N. Engl. J. Med. 2019; 380: 33-44
- Metformin improves healthspan and lifespan in mice.Nat. Commun. 2013; 4: 2192
- Impact of caloric restriction on health and survival in rhesus monkeys from the NIA study.Nature. 2012; 489: 318-321
- Impact of intermittent fasting on health and disease processes.Ageing Res. Rev. 2017; 39: 46-58
- Protein and amino acid restriction, aging and disease: from yeast to humans.Trends Endocrinol. Metab. 2014; 25: 558-566
- Effects of sex, strain, and energy intake on hallmarks of aging in mice.Cell Metab. 2016; 23: 1093-1112
- The 2015 US dietary guidelines: lifting the ban on total dietary fat.JAMA. 2015; 313: 2421-2422
- Ketogenic diet reduces midlife mortality and improves memory in aging mice.Cell Metab. 2017; 26: 547-557.e8
- The phytochemical, EGCG, extends lifespan by reducing liver and kidney function damage and improving age-associated inflammation and oxidative stress in healthy rats.Aging Cell. 2013; 12: 1041-1049
- omega-6 polyunsaturated fatty acids extend life span through the activation of autophagy.Genes Dev. 2013; 27: 429-440
- Survival of tissue-resident memory T cells requires exogenous lipid uptake and metabolism.Nature. 2017; 543: 252-256
- Linking lipid metabolism to chromatin regulation in aging.Trends Cell Biol. 2019; 29: 97-116
- FFA-induced hepatic insulin resistance in vivo is mediated by PKCδ, NADPH oxidase, and oxidative stress.Am. J. Physiol. Endocrinol. Metab. 2014; 307: E34-E46
- The PRIDE database and related tools and resources in 2019: improving support for quantification data.Nucleic Acids Res. 2019; 47: D442-D450
- Diet and aging.Cell Metab. 2008; 8: 99-104
- The omega-3 fatty acid alpha-linolenic acid extends Caenorhabditis elegans lifespan via NHR-49/PPARalpha and oxidation to oxylipins.Aging Cell. 2017; 16: 1125-1135
- Germline signals deploy NHR-49 to modulate fatty-acid beta-oxidation and desaturation in somatic tissues of C. elegans.PLoS Genet. 2014; 10: e1004829
- Modulation of longevity and tissue homeostasis by the Drosophila PGC-1 homolog.Cell Metab. 2011; 14: 623-634
- A ketogenic diet extends longevity and healthspan in adult mice.Cell Metab. 2018; 27: 1156
- Extension of mouse lifespan by overexpression of catalase.Age (Dordr.). 2006; 28: 209-218
- CD36 coordinates NLRP3 inflammasome activation by facilitating intracellular nucleation of soluble ligands into particulate ligands in sterile inflammation.Nat. Immunol. 2013; 14: 812-820
- Suppression of oxidative stress by β-hydroxybutyrate, an endogenous histone deacetylase inhibitor.Science. 2013; 339: 211-214
- Modulation of lipid biosynthesis contributes to stress resistance and longevity of C. elegans mutants.Aging. 2011; 3: 125-147
- A novel cystine based antioxidant attenuates oxidative stress and hepatic steatosis in diet-induced obese mice.Exp. Mol. Pathol. 2011; 91: 419-428
- Meta-analysis of prospective cohort studies evaluating the association of saturated fat with cardiovascular disease.Am. J. Clin. Nutr. 2010; 91: 535-546
- The ratio of macronutrients, not caloric intake, dictates cardiometabolic health, aging, and longevity in ad libitum-Fed Mice.Cell Metab. 2014; 31: 654
- Elevation of free fatty acids induces inflammation and impairs vascular reactivity in healthy subjects.Diabetes. 2003; 52: 2882-2887
- Difference of NPY and its receptor gene expressions between obesity and obesity-resistant rats in response to high-fat diet.Horm. Metab. Res. 2007; 39: 262-267
- Fat metabolism links germline stem cells and longevity in C. elegans.Science. 2008; 322: 957-960
- IQuant: an automated pipeline for quantitative proteomics based upon isobaric tags.Proteomics. 2014; 14: 2280-2285
- Dietary fats and cardiometabolic disease: mechanisms and effects on risk factors and outcomes.Nat. Rev. Cardiol. 2019; 16: 581-601
- Every-other-day feeding extends lifespan but fails to delay many symptoms of aging in mice.Nat. Commun. 2017; 8: 155
- The ketone metabolite beta-hydroxybutyrate blocks NLRP3 inflammasome-mediated inflammatory disease.Nat. Med. 2015; 21: 263-269
- PPAR-gamma and aging: one link through klotho?.Kidney Int. 2008; 74: 702-704
Article info
Publication history
Identification
Copyright
User license
Elsevier user license |
Permitted
For non-commercial purposes:
- Read, print & download
- Text & data mine
- Translate the article
Not Permitted
- Reuse portions or extracts from the article in other works
- Redistribute or republish the final article
- Sell or re-use for commercial purposes
Elsevier's open access license policy
ScienceDirect
Access this article on ScienceDirectFigures
- Graphical Abstract
- Figure 1IHF prolongs lifespan and delays tumor occurrence and effects on energy metabolic profiles in rats
- Figure 2IHF effects on blood biochemistry variables of glucose, lipids, liver/kidney function, oxidative stress, and inflammation
- Figure 3IHF effects on free fatty acid profiles in serum, liver, kidney, and muscle
- Figure 4Liver proteomic changes further reflect the improvement of oxidative stress, inflammation, and total FFA metabolism upon IHF
- Figure 5Liver proteomic changes further identified PPRC1 as a candidate target
- Figure 6Decreased PA upregulates PPRC1 involved in IHF-mediated change in lifespan, oxidative stress, and inflammation
- Figure 7Decreased PA upregulates PPRC1, contributing to IHF-dependent changes in lifespan, oxidative stress, and inflammation through PPARG