- Journal List
- HHS Author Manuscripts
- PMC7382945

Deletion of Nrf2 Shortens Lifespan in C57BL6/J Male Mice but does not Alter the Health and Survival Benefits of Caloric Restriction
Laura C.D. Pomatto
1. Translational Gerontology Branch, National Institute on Aging, National Institutes of Health, Baltimore, MD 21224, USA.
2. National Institute on General Medical Sciences, National Institutes of Health, Bethesda, MD 20892, USA.
Theresa Dill
1. Translational Gerontology Branch, National Institute on Aging, National Institutes of Health, Baltimore, MD 21224, USA.
Bethany Carboneau
1. Translational Gerontology Branch, National Institute on Aging, National Institutes of Health, Baltimore, MD 21224, USA.
Sophia Levan
1. Translational Gerontology Branch, National Institute on Aging, National Institutes of Health, Baltimore, MD 21224, USA.
Jonathan Kato
1. Translational Gerontology Branch, National Institute on Aging, National Institutes of Health, Baltimore, MD 21224, USA.
Evi M. Mercken
1. Translational Gerontology Branch, National Institute on Aging, National Institutes of Health, Baltimore, MD 21224, USA.
Kevin J. Pearson
1. Translational Gerontology Branch, National Institute on Aging, National Institutes of Health, Baltimore, MD 21224, USA.
Michel Bernier
1. Translational Gerontology Branch, National Institute on Aging, National Institutes of Health, Baltimore, MD 21224, USA.
Rafael de Cabo
1. Translational Gerontology Branch, National Institute on Aging, National Institutes of Health, Baltimore, MD 21224, USA.
Author Contributions
All experiments were designed by K.J.P., E.M.M., and R.d.C. Lifespan and whole-body experiments were carried out by T.M.W. Behavioral tests were performed by T.M.W, B.A.C, and S.R.L. Biochemical analyses were carried out by L.C.D.P and J.K. Data interpretation was conducted by L.C.D.P, M.B., and R.d.C. The manuscript was written by L.C.D.P, M.B., and R.d.C.Associated Data
Abstract
Caloric restriction (CR) is the leading non-pharmaceutical dietary intervention to improve health- and lifespan in most model organisms. A wide array of cellular pathways is induced in response to CR and CR-mimetics, including the transcriptional activator Nuclear factor erythroid-2-related factor 2 (Nrf2), which is essential in the upregulation of multiple stress-responsive and mitochondrial enzymes. Nrf2 is necessary in tumor protection but is not essential for the lifespan extending properties of CR in outbred mice. Here, we sought to study Nrf2-knockout (KO) mice and littermate controls in male C57BL6/J, an inbred mouse strain. Deletion of Nrf2 resulted in shortened lifespan compared to littermate controls only under ad libitum conditions. CR-mediated lifespan extension and physical performance improvements did not require Nrf2. Metabolic and protein homeostasis and activation of tissue-specific cytoprotective proteins were dependent on Nrf2 expression. These results highlight an important contribution of Nrf2 for normal lifespan and stress response.
Introduction
Caloric restriction (CR), defined as chronic daily reduction in total caloric intake in the absence of malnutrition, is a robust and consistent non-pharmaceutical approach for lifespan extension (1) by delaying age-associated diseases (2) and protecting against chemically-induced cancers (3). Multiple cellular pathways are responsive to CR, including the nutrient-sensing/insulin/IGF-1 signaling network (4), chromatin remodeling (5), upregulation of cellular autophagy pathways (6), as well as improvement in proteostasis and stress resistance (7). These pathways, all of which work together to promote metabolic reprogramming (8, 9), enable an organism to better adapt and cope with various forms of stress, and ultimately delaying disease onset.
Activation of the transcription factor Nrf2 (nuclear factor erythroid 2-related factor 2) represents another key response to CR (10). During periods of cellular, environmental, and oxidative stress and exposure to xenobiotics, Nrf2 dissociates from its cytosolic partner, Keap1, enabling its nuclear translocation and subsequent binding to antioxidant response elements (AREs) located upstream of stress-protective gene promoters (11). Nrf2 has been identified for its role in the upregulation of multiple phase II detoxification and cytoprotective enzymes, including NAD(P)H:quinone oxidoreductase 1 (NQO1), heme oxygenase-1 (HO-1), and subunits of the 20S proteasome. The loss of Nrf2 either as a result of age (12) or genetic manipulation (13) leads to deleterious consequences toward stress resistance and lifespan shortening in worms (14), flies (15–17), and outbred mice (18). However, the importance of Nrf2 in metabolic stress is not well understood. We have previously shown that CR-mediated lifespan extension does not require Nrf2, (3). More work is needed to elucidate the interplay between Nrf2 and different metabolic perturbations such as too few (CR) or too many (obesogenic diet) calories.
CR prevents the age-associated decline in Nrf2 target genes (19). Nrf2 is activated following a transient, small increase in oxidant signaling in response to prolonged fasting or chronic CR (20), which, in turn, confers many positive outcomes of CR, including anti-cancer protection (3). Although redundant mechanisms regulate critical facets of the pleiotropic actions of CR, the loss of Nrf2 is most deleterious when faced with challenges of oxidative stress linked to age-associated diseases (21, 22) and cancer (3). We have previously reported that Nrf2 is essential for the anticarcinogenic effects of CR in an outbred strain of mice (3), but dispensable for other hallmarks of CR, including increased insulin sensitivity and lifespan extension. These findings identified a specific molecular pathway that dissociated the pro-longevity and anticarcinogenic effects of CR. Although the work of Pearson et al. represented an important step forward in terms of our ability to discern the molecular mechanisms that drive specific phenotypes associated with CR, there were caveats that have limited the impact of the study, namely the use of ICR outbred mice in which the response to CR has not been well established (23, 24), and the lack of wild-type littermates being subjected to ad libitum (AL) and CR diets for the survival study. Here, we aimed to understand whether the genetic loss of Nrf2 would deleteriously impact CR-mediated longevity in the C57BL6/J inbred male mice. To this end, in-depth assessment of the impact of Nrf2 depletion on both the immediate response to CR and long-term metabolic adaptations associated with this regimen was performed.
Methods:
Animals and Diet
Nrf2−/− knockout (KO) mice on C57BL6/J (B6) background were obtained from The Jackson Laboratories (Bar Harbor, ME). Nrf2-KO females were crossed to B6 males to generate heterozygotes, which were subsequently crossed to generate Nrf2−/− KO and wild-type (WT) littermates. Mice were bred in-house at the National Institute on Aging (NIA) with litters being maintained with no more than 8 pups to reduce confounding effects of litter overcrowding (25). Mice were housed in cages of 3–4 with continuous water access at the Gerontology Research Center (Baltimore, MD). Mice had free access to house chow (NIH-31 diet; #T.7917.15, Envigo, Indianapolis, IN) either at ad libitum (AL) or 30% caloric restriction (CR). At baseline, there was no difference in bodyweight and mice were randomly assigned to AL or CR treatment groups. To achieve desired reduction in food intake, an incremental step-down (10% per week) was initiated until 30% CR was obtained. Body weight and food consumption were recorded on a biweekly basis. AL mice received their food in the hopper, while CR mice received their daily allotment of food between 6:30–8:30 am, which was placed in each corner of the cage. Animal rooms were maintained at 20–22°C with 30–70% relative humidity and a 12-hour light/dark cycle. All animal protocols were approved by the Animal Care and Use Committee (352-TGB-2019) of the NIA. Please see supplemental methods for details regarding animals treated with a high-fat diet (HFD).
Survival Study
Animals were inspected at least twice daily for health issues and animal deaths were recorded. Moribund animals were euthanized, with the criteria based on an independent veterinary assessment according to AAALAC guidelines. Survival curves were plotted using the Kaplan-Meier method using GraphPad Prism V7 (GraphPad Software, La Jolla, CA). The number of animals at the start of the study was: WT AL: n = 41, WT CR: n = 39, KO AL: n = 40, KO CR; n = 40. A total of 4 animals were censored in the life span study (KO CR: n = 3, WT AL: n = 1).
Body Composition
The amounts of lean, fat, and fluid mass in live mice were acquired by nuclear magnetic resonance (NMR) using the Minispec LF90 (Bruker Optics, Billerica, MA).
Serum Markers and Glucose Measurements
Fasted blood glucose was measured using Ascensia Elite glucose meter (Bayer, Mishawaka, IN). Insulin was measured using an enzyme-linked immunosorbent assay (ELISA, Crystal Chem, Inc., Downers Grove, IL) according to manufacturer’s instructions. For the oral glucose tolerance test (OGTT), animals were fasted overnight before receiving a dose of 2 g/kg of glucose (Sigma-Aldrich, St. Louis, MO) by gavage. Blood glucose was measured at baseline (t=0) and at 15, 30, 60, and 120 min after administration of glucose. The number of animals per treatment group are described in Supplemental Table 2.
HOMA Calculation
To calculate insulin resistance, fasted glucose and insulin values were used in the HOMA2-IR Calculator software available from the Oxford Center for Diabetes, Endocrinology and Metabolism, Diabetes Trials Unit: (http://www.dtu.ox.ac.uk).
Metabolic Assessment
Metabolic measurements and locomotor activity were assessed in an open-circuit indirect calorimeter using the Columbus Instruments Oxymax system (CLAMS; Columbus, OH) as previously described (26). The number of animals per treatment are presented in Supplemental Table 2.
Behavioral and Physical Performance Tests
Details regarding specific behavioral physical tests are presented in Supplemental Procedures. The number of animals used per test are presented in Supplemental Table 2.
Tissue Collection
Mice were euthanized by CO2 inhalation, followed with cervical dislocation and tissues were rapidly collected and snap frozen in liquid nitrogen and stored at −80°C for long-term storage. At 32 months, 4 mice per diet and genotype were sacrificed in the morning. On the day of the sacrifice, mice remained on their normal feeding cycles: that is mice on CR did not receive their daily ration, while AL mice could eat until sacrifice.
Gene Expression
Quantitative RT-PCR was used according to standard procedures. Full methodological details are described in Supplemental Procedures (n = 4 per treatment group).
Immunoblotting
Mouse tissues (heart, kidney, spleen) were homogenized and tissue extracts were resolved by SDS-polyacrylamide gel electrophoresis followed by immunoblotting to detect specific proteins according to standard procedures as described in Supplementary Material. The primary antibodies used in the study are listed in Supplemental Table 7 (n = 4 per treatment group).
Statistics
Results are represented as mean ± standard error of the mean (SEM). Two-way ANOVA was utilized for all comparisons, unless otherwise stated, with p value of ≤ 0.05 considered statistically significant. Median survival (defined as the 50th percentile of mice still alive) during lifespan studies was provided by Kaplan-Meier curves while the log-rank test allowed a statistical comparison of experimental groups.
Results
CR increases lifespan and improves physiological markers of health in Nrf2-KO mice
We determined the effect of Nrf2 deletion on longevity and response to CR. Starting at 30–40 weeks of age, Nrf2-KO and WT littermate male mice were randomized to either an ad libitum (AL) diet or 30% CR for the duration of their life. A 11.6% decrease in the lifespan of AL-fed mice lacking Nrf2 was observed compared to their WT littermates [logRank Mantel-Cox analysis; p < 0.001] (Figure 1A). WT mice on CR exhibited a 4.4% increase in lifespan (p = 0.012) (SI Figure 1A), while CR-fed Nrf2-KO mice displayed a 15.6% lifespan extension (p < 0.0001) (Figure 1B), becoming indistinguishable from WT littermates on CR (SI Figure 1B).
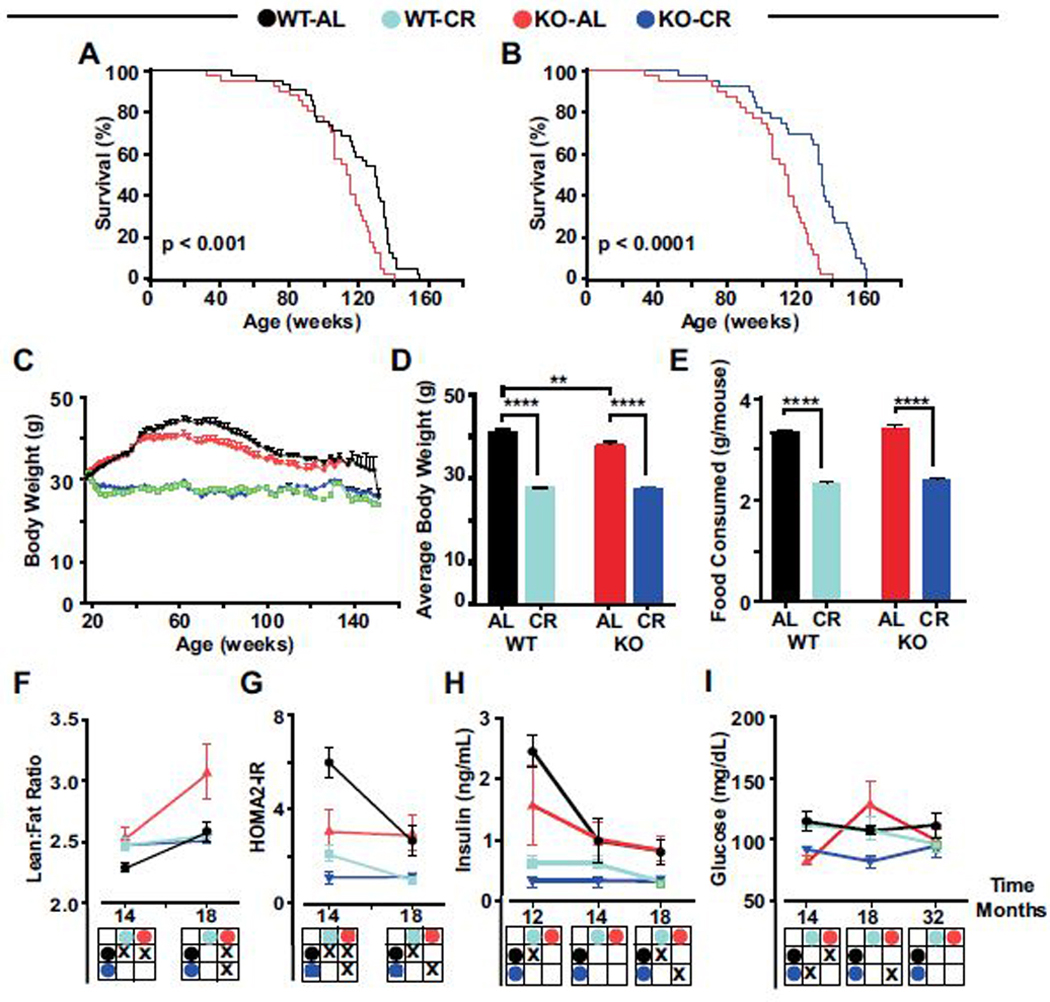
Kaplan-Meier survival curves of (A) Nrf2-KO mice and wild-type (WT) littermates under ad libitum (AL) conditions and (B) Nrf2-KO mice either on AL or 30% CR. (C) Bodyweight of WT and Nrf2-KO mice fed AL or CR over the lifespan. (D) Average bodyweight. (E) Average food consumption in Nrf2-KO mice and WT littermates on AL or CR. (F) Lean-to-fat ratio was determined by nuclear magnetic resonance (NMR) at 14 and 18 months of age. (G) HOMA2-IR at 14 and 18 months. (H) Insulin levels at 12, 14, and 18 months. (I) Fasting blood glucose levels at 14, 18, and 32 months, measured after 16 h of fasting. (F-I) Two-way ANOVA was used for D-I and statistical analysis was presented as 2 × 2 matrices: X-filled square, p < 0.05; open square, not significant.
The consumption of an obesogenic diet reduces lifespan in mice (26). Here, we found Nrf2-KO mice and WT littermates on HFD had a similar reduction in lifespan (SI Figure 1C) and increased body weight compared to the standard chow-fed animals (SI Figure 1D). Thus, the effects of CR and an obesogenic diet on longevity are not mediated by Nrf2 expression.
CR has been proposed to reduce the number of pathologies responsible for shorter lifespan (27, 28). Necropsy examination revealed lower percentage of tissue enlargement and little to no pathologies in Nrf2-KO mice and WT littermates on a 30% CR diet compared to their AL-fed controls (Supplemental Table 1). Nevertheless, CR-fed Nrf2-KO mice displayed an enlargement of the liver, a highly proliferative tissue.
CR induces physiological and morphological improvements by lowering body weight while increasing lean tissue mass (19, 29). A reduction in body weight trajectory was observed in Nrf2-KO mice and WT littermates on a 30% CR diet (Figures 1C), losing on average 30% body mass compared to their AL-fed controls (Figure 1D). The average food consumption between WT and Nrf2-KO mice was comparable (Figure 1E). AL-fed Nrf2-KO mice had lower fat mass from 14 to 18 months of age, leading to a significant increase in the lean-to-fat ratio compared to mice of the same genotype on the 30% CR diet and to WT littermates fed AL (Figure 1F).
To better understand the effect of Nrf2 deletion on metabolic changes, the age-dependent impact on insulin resistance was assessed using the Homeostasis Model Assessment (HOMA2-IR), which estimates steady state beta cell function and insulin sensitivity. Nrf2-KO mice and WT littermates placed on a CR diet showed significant improvement in insulin sensitivity at 14 and 18 months of age relative to their AL-fed counterparts (Figure 1G). Regardless of genotype, mice on an AL diet showed an age-dependent decrease in fasting insulin levels, especially between 12 and 14 months of age, and further reduction was seen in response to CR (Figure 1H). A significant reduction in fasting blood glucose (FBG) levels occurred in Nrf2-KO mice fed either AL or CR at 14 months of age (Figure 1I). Consumption of a 30% CR diet resulted in a significant reduction in FBG levels in 18-month-old Nrf2-KO mice compared to AL-fed controls; however, at 32 months of age, all groups of mice had comparable FBG (Figure 1I). As we anticipated, a strain- and diet-specific improvement in glucose clearance was observed in 14month-old mice using oral glucose tolerance tests (SI Figures 1E and 1F). It remains unclear whether Nrf2 is a key player in the maintenance of metabolic homeostasis.
We next examined the role of Nrf2 in the modulation of metabolic rate under AL and CR conditions. Nrf2-KO mice and WT littermates were placed in metabolic chambers over two light and dark cycles in order to assess substrate utilization, heat production, and spontaneous locomotor activity. The respiratory exchange ratio (RER) is defined as the ratio between the production of CO2 and amount of O2 consumed: A ratio of 1.0 reflects the preferential use of carbohydrates for energy needs while a RER of 0.7 indicates utilization of stored lipids especially during fasting (30). Assessment in the changes in metabolic output was measured at 20- and 32-month-old WT and Nrf2 KO mice fed either AL or a 30% CR diet. Consistent with previous reports that CR-fed mice engage in the utilization of lipids from lipolysis of fat depots as the predominant energy sources (31), we observed a significant decrease in RER in response to CR, especially in Nrf2-KO mice during the dark cycle (SI Figures 2A and 2B). A similar effect was evident with heat production (SI Figures 2C and 2D). AL-fed WT mice showed the lowest amount of ambulatory activity during the day (SI Figure 2E), whereas Nrf2-KO mice on AL were the only group with increased activity at night, which dissipated at 32 months of age (SI Figure 2F).
We also explored the impact of high fat diet (HFD) on metabolic parameters in mice lacking Nrf2. Twelve-month-old mice of both genotypes showed the expected decrease of RER when fed an HFD, as evidenced by preferential utilization of fats as the primary energy source (SI Figure 3A and 3B). Increased heat generation, but lower spontaneous activity was observed upon consumption of the obesogenic diet (SI Figure 3C, 3D, and 3F). Of note, Nrf2-KO mice on AL exhibited significant reduction in spontaneous activity during the dark phase compared to AL WT littermates (SI Figure 3F).
Impact of CR and Nrf2 on Physical Performance
The open-field performance is a means of measuring overall activity and exploratory behavior, rotarod assess changes in motor function, while cage top and grip strength tests monitor changes in muscle strength. Here, we used both behavioral tests to evaluate the impact of age, diet, and genotype and found that the open-field performance was unresponsive to age (up to 18 months) and genetic background (Figure 2A), whereas an age-dependent decline of muscle strength was observed in AL-fed WT mice (Figure 2B). Significant reduction in muscle strength was seen only in 18-month-old Nrf2-KO mice (Figure 2B). Twenty-month-old mice on CR had marked improvement in ambulatory and exploratory activities (Figure 2C) and grip strength (Figure 2E) regardless of the genotype. However, Nrf2-KO mice on AL displayed better motor coordination and grip strength compared to age-matched WT littermates (Figure 2D, ,E).E). Lastly, at 32 months of age, both muscle strength and grip strength of CR-fed Nrf2-KO mice were significantly lower than that of WT littermates (Figure 2F and and2H)2H) and did not correlate with motor coordination (Figure 2G). Overall, Nrf2 appears to contribute to the benefits of CR in old animals.
(A) Total distance traveled in open field and (B) Cage-top latency in AL-fed Nrf2-KO mice and WT littermates at 7, 14, and 18 months of age. (C) Total distance traveled in open field. (D) Time to fall from an accelerating rotarod, and (E) Wire-hang latency in 20-month-old mice of both genotypes on either AL or 30% CR. (F) Cage-top latency. (G) Time to fall from an accelerating rotarod, and (H) Wire-hang latency at 32 months in mice on 30% CR (WT-AL and KO-AL were not measured “n.m.”). Statistical significance indicated with asterisk, * p < 0.05, ** p < 0.01, *** p < 0.001. Two-way ANOVA was used for A-E; Student’s t-test was used for F-H to determine significance.
Stress-Inducible Proteins are Responsive to Nrf2 and CR
Next, we assessed whether the loss of Nrf2 influenced the expression of multiple stress-protective proteins in mice under AL or CR. Preliminary studies confirmed the extent of Nrf2 knockdown, as evidenced by the marked reduction in Nrf2 mRNA levels in the heart, kidney and spleen of 32-month-old mice (SI Figure 4). Then, immunoblot analysis using total tissue lysates was carried out. The results illustrated a clear tissue-specific response to Nrf2 depletion, especially in the spleen, with a significant decline in the abundance of stress-protective enzymes such as 20S proteasome catalytic subunit beta 1 (20S β1), NQO1, and HO-1 (Figure 3; SI Figure 5; SI Table 8), highlighting the role of Nrf2 as modulator of innate immunity (32, 33).
Heart, kidney, and spleen lysates from 32-month-old Nrf2-KO mice and WT littermates fed AL or 30% CR were resolved by SDS-PAGE and immunoblotted for the beta1 catalytic subunit of the 20S proteasome, NAD(P)H quinone dehydrogenase 1 (NQO1), and heme oxygenase-1 (HO-1). Full blots are shown in Supplementary Figure 5 with mean and standard error of the mean indicated in Supplementary Table 9. Statistical significance is indicated with letter, a: p < 0.05, b: p < 0.01, c: p < 0.001. Color indicates the comparison, black: WT-AL; light blue: WT-CR; red: KO-AL. Two-way ANOVA and Tukey’s multiple comparison test was used to determine significance (Supplemental Table 4).
Nrf2 has a role in the targeting of proteins to mitochondria (34) and in mitochondrial homeostasis (35). Increasing mitochondrial OXPHOS function in cells requires an upregulation of the transcriptional coactivator PGC-1α (36), whose levels were significantly reduced in Nrf2-KO mice under AL (heart) and CR (heart & spleen) (Table 2; SI Figure 6). Lower levels of the Lon protease, a nuclear-encoded mitochondrial protease, were observed in the heart and spleen of CR-fed Nrf2-KO mice. Mitochondrial chaperone hsp60 levels were also depleted in the same tissues of Nrf2-KO mice vs. WT littermates, regardless of diet (Figure 4; SI Figure 6; SI Table 9). Lastly, the levels of mitochondrial-specific manganese superoxide dismutase (MnSOD) were significantly lower in the heart and spleen, but higher in the kidney of CR-fed Nrf2 KO mice (Figure 4; SI Figure 6; SI Table 9). Overall, these findings indicate that the deletion of Nrf2 has a strong impact on the heart especially with regard to the expression of genes targeted to the mitochondria.
The same tissue lysates used in SI Figure 5 were immunoblotted for PGC-1α, mitochondrial Lon protease, heat shock protein 60 (hsp60), and manganese superoxide dismutase (MnSOD). Full blots are depicted in Supplementary Figure 6 with mean and standard error of the mean indicated in Supplementary Table 9. Statistical significance is indicated with letter, a: p < 0.05, b: p < 0.01, c: p < 0.001. Color indicates the comparison, black: WT-AL; light blue: WT-CR; red: KO-AL. Two-way ANOVA and Tukey’s multiple comparison test was used to determine significance (Supplemental Table 5).
Discussion
Nrf2 is regarded as one of the main transcriptional orchestrators of the phase II detoxification system and necessary for redox homeostasis (12). Numerous studies have shown Nrf2 to play a central role in protecting against cancer development (37), and a weakening in Nrf2 signaling occurs with age (16, 38) and chronic disease (39, 40). Delay in Nrf2 dysregulation can be achieved by CR through ill-defined mechanisms (20), which may contribute to the lifespan extending properties of CR.
Our prior work in the ICR outbred mice highlighted the importance of the Nrf2 pathway, dissociating the prolongevity and anticarcinogenic effects of CR. Under CR, Nrf2 is dispensable for increased insulin sensitivity and lifespan extension, while playing an important role in mediating the protection against chemically-induced carcinogenesis (3). Here, we sought to explore in greater depth the role of Nrf2 in regulating lifespan and mediating the response to CR. To this end, a more in-depth assessment of how CR impacts longevity, metabolic regulation, and physiologic function was performed in WT and Nrf2 KO mice on a C57BL/6J background. The effects of CR have been well established in this inbred mouse strain. There is mounting evidence to suggest that the longevity and functional improvement effects of CR are a universal response, as the age of onset (41, 42), sex (29, 43), and strain (42, 44–46), as well as the level and timing of CR (29, 47, 48) all contribute to CR responsiveness (for a review (49)).
As in worms (50) and flies (16), our results show that the absence of Nrf2 was associated with decreased survival under ad libitum conditions, indicating the importance of Nrf2 to achieve a normal lifespan in mice. This finding is important because it highlights the necessary role of Nrf2 in mitigating excess accumulation of damaged macromolecules from physiological levels of reactive oxygen species. Moreover, because Nrf2 regulates multiple antioxidant pathways necessary for homeostatic balance, removal of individual downstream Nrf2 targets (e.g., MnSOD, Gpx1, or Gpx4) was found to have no negative impact on lifespan (51). Thus, we surmise that redundant or parallel stress-protective pathways, which are not activated in the absence of Nrf2, can explain the strong phenotype in Nrf2-KO mice compared to WT littermates.
Together, these findings highlight the necessity of taking a new approach in how we test one of the leading theories of aging, the ‘Oxidative Stress Theory of Aging.’ For the past three decades, much work has centered on assessing the impact of changing either the levels of oxidative stress or its organismal damage in order to determine how these alterations affect lifespan. The creation of multiple mouse lines to Nrf2-downstream targets, using DNA recombinant technology that applies either transgenic/knockout approaches, clearly demonstrate that almost all alterations in a single antioxidant gene have no effect on mouse lifespan while conferring protection against diseases (51). However, these findings highlight two important points: first, the failure to recognize the need to impose a physiological stress (metabolic, oxidative, environmental) in these genetic models in order to perturb the system out of its homeostatic range (52), and, second, the recognition that key mechanistic differences exist in the regulation of healthspan and lifespan (53).
In agreement with our earlier work (3), we found that Nrf2 is not required for CR-mediated lifespan extension. In the current study we found that the loss of Nrf2 does not magnify lifespan shortening due to chronic consumption of HFD. On the other hand, Nrf2 had an essential role in the survival of mice fed standard lab chow. The fact that Nrf2-KO mice were also resistant to the metabolic response to CR further supports the importance of metabolic adaptations leading to increased lifespan (54, 55). Contrary to previous observations showing that the loss of Nrf2 confers protection against diet-induced obesity (56, 57), our results suggest that Nrf2-KO mice are unable to mount resistance from metabolic stress caused by an obesogenic diet. Nrf2 deficiency has been reported not only to promote neuroinflammation, but also to further exacerbate it under HFD (58). Possible explanations for the difference between our study and the previous ones include their use of relative short length of treatment (maximum 12 weeks) (56, 57) and utilization of animal-derived fats (57). Thus, the Nrf2 pathway, which largely centers on protection against oxidative stress, may serve as an independent and complementary pathway to CR, and its loss may be compensated by additional cellular maintenance pathways such as autophagy (59).
Additionally, changes in body weight are consistent with prior studies conducted by Mitchell et al. and others showing that the maintenance of some adiposity or the rapid loss in fat mass during CR seems to predict lifespan benefits in mice (60, 61). Thus, the ability to maintain fat mass may partly explain the beneficial prolongevity properties of CR (29). Interestingly, unlike in mice, the NIA female rhesus monkeys preserved their body weight and fat content in response to CR, yet had shortened lifespan compared to AL-fed controls (62), suggesting the disconnect between healthspan (i.e. disease-free and functional ability) versus lifespan.
Nrf2 plays a key role in cancer suppression via activation of the antioxidant pathway (37) and prevention of malignant cell formation (37, 63). In addition, an age-dependent decline in the transient induction of Nrf2 (16, 64–67) may result in a sustained increase in basal levels of oxidative stress, which, in turn, elevates cancer risk (68–70). The ability of Nrf2 to confer protection against spontaneous tumor development could not be reproduced by CR treatment in Nrf2-KO mice (3), highlighting the convergence of these two pathways in cancer protection. Indeed, we found that the loss of Nrf2 leads to lower expression of the 20S proteasome, NQO1, and HO-1 that are involved in detoxification, proteostasis/clearance, and redox balance (12).
Mice lacking Nrf2 weighed less than their WT littermates even under ad libitum feeding, whether in C57BL/6JB (this study) or the ICR outbred strain (3). However, food consumption did not differ among the experimental groups, suggesting changes in metabolic rate between these two genotypes. We have found that Nrf2-KO mice have increased heat production, consistent with potential ‘browning’ of fat tissue. It has been recently reported that mice lacking Nrf2 showed a significant increase in energy expenditure and upregulation of UCP1 (57), a mitochondrial uncoupler which dissipates energy of glucose/lipid oxidation as heat (71). Mice deficient in Nrf2 have significantly higher lean-to-fat ratio (56, 57) and improved insulin sensitivity when fed an obesogenic diet (72). Insulin-stimulated glucose uptake and utilization generates a transient increase in reactive oxygen species due to mitochondrial substrate oxidation and associated increase in electron leak (73) in skeletal muscle and other metabolically active tissues (e.g., fat depots and the brain). Our results indicate that the beneficial effects of CR on glucose homeostasis and insulin sensitivity were maintained in Nrf2-KO mice, which is consistent with the existence of alternative, compensatory pathways triggered by the depletion in Nrf2. We postulate that the protective functions associated with Nrf2 went from key regulator of cellular resistance to oxidative stress to a more nuanced role of Nrf2-mitochondria crosstalk than previously thought.
The disconnect between healthspan and lifespan (i.e. one not guaranteeing an improvement in the other) is potentially addressed through the lens of the mitochondrial free radical theory of aging. Prior work suggests the lowering in mitochondrial reactive oxygen species generation and overall dampening of cellular oxidative stress in response to caloric restriction (74, 75). To explore the growing evidence suggests possible interplay between Nrf2 and the mitochondria (35), in part through increased expression of PGC-1α a key transcriptional regulator of mitochondrial biogenesis (76, 77), that decreases with age (78, 79) and disease (80). In addition to mitochondrial biogenesis, there is a decline in the capacity of cells to maintain mitochondrial proteostasis during aging (81). Indeed, the mitochondrial Lon protease is a key stress-inducible, degradative enzyme involved in the turnover of oxidized aconitase (82) whose activity declines in older animals (83). The maintenance of mitochondrial proteostasis depends on the expression of key chaperones including Hsp60 whose levels are controlled by the transcriptional regulator HSF1 (84). HSF1 is a versatile protein that declines with age (85). HSF1 controls distinct cytoprotective pathways and there is growing evidence to suggest an interplay between HSF1 and Nrf2 and their ability to co-regulate the expression of target genes (86), including HO-1 (87) and HSP70 (88). Here, our results show that Nrf2-KO mice have lower expression of PGC-1α in the heart and spleen, two tissues with high metabolic demands, and decreased levels of Hsp60 protein, likely due to blunted HSF1 activation. Although no significant decrease in the amount of Lon protein was observed in AL-fed Nrf2-KO mice, consumption of a CR diet led to significant reduction in Lon levels, suggesting the need for future studies evaluating Lon activity in these animals.
In summary, our study demonstrates the lack of Nrf2 involvement in the longevity and metabolic adaptations to a 30% CR diet and HFD consumption using an inbred strain. The loss of Nrf2 did not impact the ability of CR to improve motor function at 20 months of age, suggesting the activation of compensatory pathways in response to Nrf2 depletion. Changes in expression levels of stress-protective and mitochondrial-specific enzymes in multiple tissues (heart, kidney, spleen) of aged animals were found with respect to genotype (Nrf2-KO vs. WT) and diet (CR vs. AL), which could help explain, at least partly, the mechanisms by which both CR and Nrf2 contribute as pro-longevity effectors. As the role of Nrf2 in cancer prevention hints at its importance in the immune surveillance system (89), it is vital to delve further into understanding the interplay between the immune system and dysregulation of Nrf2 signaling in health and disease.
- Loss of Nrf2 results in shortened lifespan in C57BL/6J male mice.
- Calorie restriction-mediated lifespan extension is independent of Nrf2.
- Unabated positive health outcomes of calorie restriction in Nrf2-null mice
- Reduced expression of cytoprotective and mitochondrial proteins in Nrf2-null mice
Acknowledgements
This work was supported by funding from the Intramural Research Program of the National Institute on Aging/NIH. L.C.D.P. was supported by the NIH Grant #Fi2GM123963 from the National Institute of General Medical Sciences of the National Institutes of Health. Special thanks to the members of the Translational Gerontology Branch and the Comparative Medicine Section of the National Institute on Aging. We are particularly grateful to Dawn Boyer and Dawn Nines for their excellent animal care.
Abbreviations
Nrf2 | Nuclear factor erythroid-2-related factor 2 |
Keap1 | Kelch-like ECH associated protein 1 |
ARE | Antioxidant response element |
NQO1 | NAD(P)H quinone dehydrogenase 1 |
HO-1 | Heme oxygenase 1 |
OXPHOS | Oxidative phosphorylation |
PGC-1α | Peroxisome proliferator-activated receptor gamma coactivator 1-alpha |
HSP 60 | Heat shock protein 60 |
MnSOD | Manganese superoxide dismutase |
Gpx1 | Glutathione peroxidase 1 |
Gpx4 | Glutathione peroxidase 4 |
UCP1 | Uncoupling protein 1 |
HSF1 | Heat shock factor 1 |
HSP 70 | Heat shock protein 70 |
Footnotes
Conflict of interest
None to declare
Publisher's Disclaimer: This is a PDF file of an unedited manuscript that has been accepted for publication. As a service to our customers we are providing this early version of the manuscript. The manuscript will undergo copyediting, typesetting, and review of the resulting proof before it is published in its final form. Please note that during the production process errors may be discovered which could affect the content, and all legal disclaimers that apply to the journal pertain.